THE EFFECTIVENESS OF RHIZOBACTERIA AS BIOPROTECTANTS TO MITIGATE FUSARIUM WILT DISEASE AND AS BIOSTIMULANTS TO IMPROVE THE GROWTH OF CHILI (Capsicum annuum)
on
The Effectiveness of Rhizobacteria as Bioprotectants to Mitigate Fusarium Wilt Disease and as Biostimulants oo Improve
the Growth of Chili (Capsicum annuum)
Khalimi, K, Suputra, IPW, Wirya, A.S, Innosensia, NLPC.
THE EFFECTIVENESS OF RHIZOBACTERIA AS BIOPROTECTANTS
TO MITIGATE FUSARIUM WILT DISEASE AND AS BIOSTIMULANTS
TO IMPROVE THE GROWTH OF CHILI (Capsicum annuum)
Khamdan Khalimi1*, I Putu Wirya Suputra1, Alit Susanta Wirya1, Ni Luh Putu Citra Innosensia2
-
1 Department of Agroecotechnology, Faculty of Agriculture, Udayana University, Badung, Bali, Indonesia.
-
2 Department of Symbiotic Science of Environment and Natural Resources, United Graduate School of Agriculture Science, Tokyo University of Agriculture and Technology, Tokyo, Japan. *Corresponding Author: [email protected]
ABSTRACT
The aims of this study were to examine the potential of Rhizobacteria as bioprotectants and biostimulants on chili. The potential as bioprotectants was tested by the ability of these Rhizobacteria to inhibit the growth of the pathogenic fungus Fusarium oxysporum f.sp. capsici in vitro. Moreover, as biostimulants was tested by the ability of these rhizobacteria to produce IAA compounds and their ability to increase the plant growth parameter of chili. Four rhizobacteria were challenged for antagonistic activity against F. oxysporum f.sp. capsici. Chili seeds in this experiment were soaked in four different Rhizobacteria suspension for 15 minutes. For control, seeds were soaked with sterile water instead of Rhizobacteria suspension. Results of this experiment showed that four isolates of Rhizobacteria showed strong inhibitory activity against F. oxysporum f.sp. capsici on PDA medium. Percentage of inhibitory activity varied from 77,33% to 89,79%. The application of four isolates recorded significantly increased the plant growth parameters of chili. The plant height, number of leaves, leaf area, root length, shoot dry mass, root dry
Received:
27 September 2022
mass, and chlorophyll content on treated plants significantly higher than those of untreated control plants according to the Duncan’s multiple range
Accepted:
test (P<0.05%). These results indicate that the tested Rhizobacteria can be
2 October 2022
used as bioprotectants because they can inhibit the growth of F.
Published:
oxysporum f.sp. capsici and simultaneously as a biostimulant for the
4 Oktober 2022
ability to produce IAA compounds and stimulate the growth of chili.
Keywords: Bioprotectant, biostimulant, IAA, inhibitory,rhizobacteria
INTRODUCTION
Chili is an important and popular commodity in Indonesia which has a high economic value. This spice is one of the most widely used for household consumption, industry, and in food processing (Ismawati, 2016). However, the average productivity of chili in Indonesia is low. According to the Central Bureau of Statistics (2022), in 2021 national chili production in Indonesia decreased by 8.09% compared to the production in 2020. The national chili production decreased to 1.39 million tons in 2021 while in 2020 the national chili production was 1.5 million tons. One of the factors that lower chili production in Indonesia is because of fusarium wilt disease invasion.
Wilt disease on chili commonly caused by the fungal infection Fusarium oxysporum f. sp capsici. The use of synthetic fungicides to control F. oxysporum f.sp. capsici has been widely practiced and proven less effective in controlling this fungus. Besides, an excessive amount of applied synthetic fungicides lead to severe environmental pollution. One of the efforts that become a moral responsibility for us to reduce the use of synthetic fungicides is to utilize a more environmentally friendly approach like Rhizobacteria that potentially act as bioprotectants and biostimulants. As bioprotectants Rhizobacteria can produce substances that have biological activity as antimicrobials that act to protect plants from pathogents. While as biostimulants, Rhizobacteria can increase plant growth because they produce substances that have biological activity as growth promoters.
The purpose of this study was to examine the potential of Rhizobacteria as
bioprotectants by challenging their ability to inhibit the growth of F. oxysporum f.sp. capsici in vitro and to examine the potential of rhizobacteria as biostimulants by testing their ability to produce IAA compounds and mediated the growth of chili.
MATERIALS AND METHOD Time and Place of Research
This research was conducted at the Plant Protection Laboratory, Faculty of Agriculture, Udayana University from April to August 2022. The materials used in this study were 4 Rhizobacteria: Pantoea agglomerans TR78, Klebsiella pneumoniae KC66, Klebsiella pneumoniae SW04, Stenotrophomonas maltophilia KC29, PDA medium (4g Potato extract, 20 g sugar, 15g agar, Oxoid ltd., CM139) and chili seeds (Bara, East-West Seed). Chlorophyllmeter SPAD-502, Minolta Japan was used to measured the leaves chlorophill content.
The Potential of Rhizobacteria as Bioprotectant
The potential of Rhizobacteria as bioprotectants was examined by testing their ability to inhibit the growth of the pathogenic fungus Fusarium oxysporum f.sp. capsici in vitro. Inhibition testing was carried out based on the method of Mayadianti et al. 2020, namely first, preparation of growth media by pouring 10 ml of PDA media on a Petri dish. Furthermore, the fungus F. oxysporum f.sp. capsici was inoculated on PDA media, in the middle of the Petri dish, then each rhizobacteria, namely P. agglomerans TR78, K. pneumoniae KC66, K. pneumoniae SW04, and S. maltophilia KC29 were inoculated in 4 positions flanking the fungus, each 2 cm away from the fungal colony.
Each treatment was repeated 5 times. The design used in this study was a completely randomized design (CRD) with 5 treatments and 5 replicates. The treatments were the control fungus F. oxysporum f.sp. capsici (KTL0), rhizobacteria P. agglomerans TR78 + F. oxysporum f.sp. capsici (TR78), rhizobacteria K. pneumoniae KC66 + F. oxysporum f.sp. capsici (KC66), rhizobacteria K. pneumoniae SW04 + F.
oxysporum f.sp. capsici (SW04), and rhizobacteria S. maltophilia KC29 + F. oxysporum f.sp. capsici (KC29). The growth rate of fungal colonies was determined using the equation (1). Determination of the percentage of rhizobacterial inhibition against fungal growth is determined by equation (2)
Colony area at last observation
Colony growth rate =
Time interval
…………………..……………………….(1)
Inhibition =
Control colony area - Colony area of treatment
Control colony area
× 100%
(2)
Test of Rizobacterial Potential as Biostimulant
The potential test of rhizobacteria as biostimulants was conducted by testing the ability of rhizobacteria to produce IAA compounds and testing their ability to increase the growth of chili plants. The test of the ability of rhizobacteria to produce IAA was conducted by colorimetric method based on Wahyudi et al. (2011). Rhizobacteria were grown for 48 hours in Nutrient Broth medium added with 1 mM L-tryptophan. The rhizobacterial culture was centrifuged at 1610 x g for 30 min, then the supernatant was separated from the bacterial sediment and filtered with Millipore filter paper (0.45 mm). Furthermore, IAA content in the filtrate was detected using Salkowski reagent (1 ml 0.5 M FeCl3 and 49 ml 35% HClO4). A total of 1 ml of rhizobacterial filtrate was put into a 2 ml eppendorf tube and then 1 ml of Salkowski reagent was added. The eppendorf tube containing the solution was incubated in a dark room at room temperature for 30 minutes. A change in the color of the solution in the eppendorf
tube to pink indicates that the rhizobacteria produce IAA.
Test the ability of rhizobacteria in increasing the growth of chili plants was carried out by invigorating chili seeds with 2% suspension of each rhizobacteria. The 2% concentration was obtained from 2 ml of culture in PPDC media (5 g potato extract, 5 g peptone, 10 g dextrose, 1000 ml Coconut water) per 100 ml of water. The design used in this study was a randomized group design (RAK) with 5 treatments and 5 replicates. The treatments were control (KTRL); seed invigoration with P. agglomerans TR78 suspension (PAGS); seed invigoration with K. pneumoniae KC66 suspension (KLPE); seed invigoration with K. pneumoniae SW04 suspension (KLPN); seed invigoration with S. maltophilia KC29 suspension (STMA). Each replicate consisted of 10 plants. Seeds were then planted in pots that had been filled with planting media. Growth parameters observed were plant height, number of leaves, root dry weight, crown dry weight, root length, leaf area, and leaf chlorophyll content. Measurement of leaf chlorophyll
content (SPAD unit) was determined with Chlorophyllmeter SPAD-502.
RESULTS AND DISCUSSION
Test of Rizobacterial Potential as Bioprotectant
The test results of the ability of rhizobacteria to inhibit the growth of F. oxysporum f.sp. capsici in vitro showed that rhizobacteria were able to inhibit the growth of F. oxysporum f.sp. capsici fungal colonies with the percentage of inhibition ranging from 77.33% to 89.79% (Table 1). The area
eISSN: 2655-9994 pISSN: 2303-3371 https://doi.org/10.24843/IJBB.2022.v10.i01.p04
of fungal colonies in the TR78 treatment amounted to 285.77 mm2 with a percentage inhibition of 77.33% when compared to the control. While the colony area in the SW04 treatment amounted to 267.82 mm2 with a percentage inhibition of 78.76%, the colony area in the KC29 treatment amounted to 188.21 mm2 with a percentage inhibition of 85.07%, and the colony area in the KC66 treatment amounted to 128.79 mm2 with the highest percentage inhibition of 89.79% when compared to the control.
Table 1. The results of the rhizobacterial ability test in inhibiting the growth of the fungus F.
oxysporum f.sp. capsici in vitro
Treatments |
Fungal colony area (mm2) |
Colony growth rate (mm2/day) |
Inhibition (%) |
KTL0 |
1261,01 a* |
180,14 a |
- |
TR78 |
285,77 b |
40,82 b |
77,33 |
KC66 |
128,79 d |
18,39 d |
89,79 |
SW04 |
267,82 b |
38,26 b |
78,76 |
KC29 |
188,21 c |
26,88 c |
85,07 |
* Values in the same column followed by the same letter are not significantly different (p>0.05) according to Duncan's Multiple Range Test at the 5% level.
Fungal colonies of F. oxysporum f.sp. capsici in the control treatment grew normally with a colony growth rate of 180.14 mm2/day (Figure 1 and Table 1). While the fungal colonies in the rhizobacterial treatment grew abnormally or inhibited. This can be seen in the low value of fungal colony area which is a manifestation of fungal growth. The lower the area value of fungal colonies, the higher
the inhibitory value of rhizobacteria against fungi. Fungal growth in the rhizobacterial treatment was abnormal due to the presence of antifungal compounds produced by rhizobacteria through the mechanism of antibiosis. Fungal growth in the rhizobacterial treatment was inhibited with colony growth rates ranging from 40.82 mm2/day to 18.39 mm2/day.
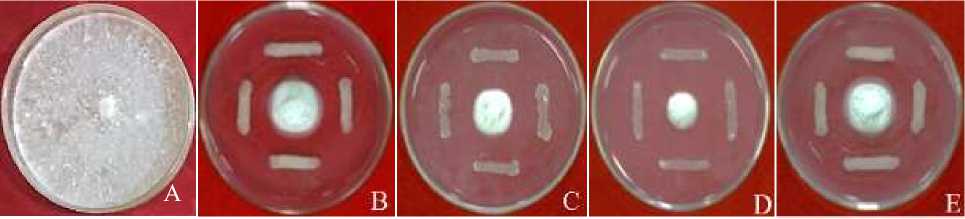
Figure 1. Test the ability of rhizobacteria in inhibiting the growth of fungus F. oxysporum f.sp.
capsici in vitro A. KTL0 treatment; B. TR78 treatment; C. KC29 treatment;
D. KC66 treatment; and E. SW04 treatment
Test of Rizobacterial Potential as Biostimulant
The results of colorimetric tests on rhizobacterial cultures showed that 4 tested rhizobacterial cultures experienced a color change from clear to pink (Figure 2). Meudt and Gaines (1967) reported that Salkowski reagent contains 35% HClO4 and 10 mM FeCl3 and when reacted with rhizobacterial cultures containing IAA there will be a color change from colorless to pink indicating that IAA is oxidized by HClO4. Rahman et al. (2010) reported that the color change to pink indicates that IAA is oxidized by HClO4 and then oxidized IAA reacts with FeCl3 to form tris-(indole-3-acetato) iron (III) complex.
Kamnev et al. (2001) reported that the compound tris-(indole-3-acetato) iron (III) complex has the molecular formula FeC30H24N3O6 or Fe[(C8H6N)-CH2COO]3). Meudt and Gaines (1967) reported that IAA, 5-Hydroxyindole-3-acetic acid, and indole-3-acetamide compounds will be pink when reacted with Salkowski reagent. Colorimetric method can be used to determine the concentration of IAA, where the pinker the test solution, the higher the concentration of IAA in the solution. This is because one molecule of Fe will bind three molecules of IAA to form a tris-(indole-3-acetato) iron (III) complex.
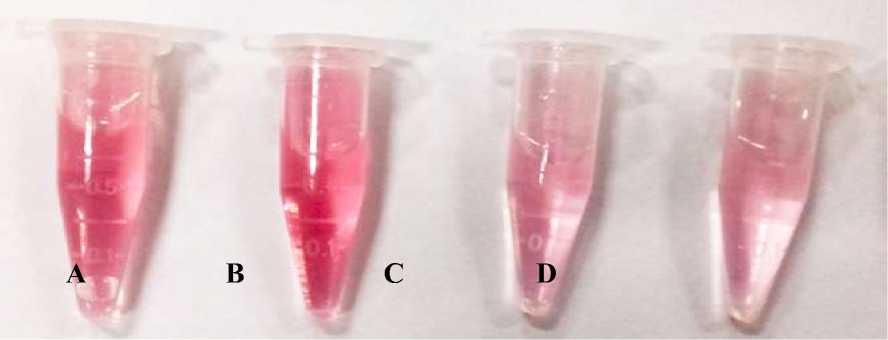
Figure 2. Colorimetric test results on rhizobacterial cultures. A. Filtrate of S. maltophilia KC29; B. Filtrate of P. agglomerans TR78; C. Filtrate of K. pneumoniae KC66; D. Filtrate of K. pneumoniae SW04.
The test results of the ability of rhizobacteria in increasing the growth of chili plants showed that the four tested
rhizobacteria were able to increase the growth parameters of chili plants (Table 2). The rhizobacterial treatments were able to
increase plant height ranging from 30.21% to 42.55% when compared to the control. PAGS treatment increased plant height by 42.55%, KLPE treatment by 34.04%, KLPN treatment by 36.59%, STMA treatment by 30.21% when compared to the control. Some researchers also reported similar results that rhizobacteria can increase plant height. Park et al. (2015) reported that the treatment of B. amyloliquefaciens IN937a, B. cereus BS107, B. pumilus INR7, B. subtilis GB03 on K. daigremontiana plants was able to increase plant height by 20.0% when compared to the control. Rathaur et al. (2012) reported that
the treatment of rhizobacterial isolate PG9 increased the plant height of W. somnifera by 22.76% when compared to the control. Asghar et al. (2002) reported that the treatment of B. juncea plant seeds with rhizobacterial isolate S54 was able to increase plant height by 56.54% when compared to the control. Asghar et al. (2004) reported that treatment of Brassica napus plant seeds with rhizobacterial isolate S58 which produced 24.03 µg ml-1 IAA increased plant height by 39.86% when compared to the control.
Table 2. The results of the rhizobacterial ability test in increasing the growth parameters of chili
plants
Treatments Plant |
Leaves |
Leaves |
Root dry |
Shoot dry |
Chlorophyll |
Root length |
heaight |
number |
area |
mass (g) |
mass (g) |
content |
(cm) |
(cm) |
(helai) |
(cm2) |
(SPAD) |
KTRL |
23,5 a |
10,33 a |
29,40 a |
0,206 a |
0,740 a |
35,83 a |
28,77 a |
PAGS |
33,5 b |
13,67 a |
33,85 b |
0,547 c |
1,693 b |
43,91 b |
23,67 b |
KLPE |
31,5 b |
12,98 a |
31,76 b |
0,316 b |
1,137 b |
41,34 b |
26,33 b |
KLPN |
32,1 b |
12,24 a |
33,38 b |
0,322 b |
1,150 b |
42,67 b |
29,50 a |
STMA |
30,6 b |
12,54 a |
31,47 b |
0,351 b |
1,173 b |
42,87 b |
25,23 b |
Note: values followed by different letters in the same column indicate significantly different
based on Duncan's test (P < 0.05).
Rhizobacteria treatment was able to increase the number of leaves ranging from 18.48% to 32.33% when compared to the control. PAGS treatment increased plant height by 32.33%, KLPE treatment by 25.65%, KLPN treatment by 18.48%, STMA treatment by 21.39% when compared to the control. Some researchers also reported similar results that rhizobacteria can increase the number of leaves. Rathaur et al. (2012) reported that the treatment of rhizobacterial isolate PG10 increased the number of leaves of Withania somnifera plants by 21.62%
when compared to the control. Rajan and Radhakrishna (2013) reported that the treatment of Klebsiella sp. LEE19 which produced IAA 212.3 µg ml-1 increased the number of leaves of Hibiscus rosasinensis plants by 334.28% when compared to the control. Yaqoob et al. (2013) reported that the treatment of Azospirillum sp. G1 producing IAA in Triticum aestivum plants increased the number of leaves by 75% when compared to the control. Gusmaini et al. (2013) reported that the treatment of bacterial isolate 5 MD on Andrographis
paniculata plants increased the number of leaves by 116.67% when compared to the control. Sudharani et al. (2014) reported that the treatment of Azotobacter chroococcum Az on cabbage plants increased the number of leaves by 25.68% when compared to the control.
Rhizobacterial treatments were able to increase leaf area ranging from 7.04% to 15.13% when compared to the control. PAGS treatment increased leaf area by 15.13%, KLPE treatment by 8.02%, KLPN treatment by 13.53%, STMA treatment by 7.04% when compared to the control. Some researchers also reported similar results that rhizobacteria can increase leaf area. Sivasankari et al. (2014) reported that the application of 5 ml suspension of B. cereus suspension with the addition of 2 mg ml-1 tryptophan in the media increased the leaf area index of cowpea plants aged 15 HST by 127.45% when compared to the treatment of 5 ml of B. cereus suspension without the addition of tryptophan. cereus suspension without the addition of tryptophan. Gholami et al. (2009) reported that seed coating treatment of corn seeds with a suspension of P. putida DSM291 with a population density of 108 cfu ml-1 increased leaf area by 190.56% when compared to the control. Karakurt and Aslantas (2010) reported that spraying a suspension of B. subtilis OSU-142 with a population density of 109 cfu ml-1 at first bloom and full bloom of apple plants increased leaf area by 13.71% when compared to the control. Turan et al. (2014) reported that treatment of cabbage seeds with Pantoea agglomerans RK-92 increased leaf area by 27.25% when compared to the control.
Rhizobacterial treatments were able to increase root dry weight ranging from 53.39% to 165.53% when compared to the control. PAGS treatment increased root dry weight by 165.53%, KLPE treatment by 53.39%, KLPN treatment by 56.31%, STMA treatment by 70.38% when compared to the control. Some researchers also reported similar results that rhizobacteria can increase root dry weight. Qi et al. (2016) reported that six strains of B. thuringiensis increased the dry weight of tomato plants ranging from 35.3% to 201.9% when compared to the control. Reetha et al. (2014) reported that B. subtilis which produces IAA 12.67 µg/ml on Nutrient Broth media increased the dry weight of onion plant roots by 15.47% when compared to the control. While Zahid et al. (2015) reported that the treatment of bacterial isolate HJR3 which produces IAA increased the dry weight of roots by 211.11% when compared to the control. Swain et al. (2007) reported that B. subtilis CM4 which can produce IAA 2.1 mg/l with the addition of 0.1 g/l tryptophan in Nutrient broth increased the dry weight of roots and crowns of Dioscorea rotundata plants by 254.54% and 291.67% when compared to the control. Khalid et al. (2004) reported that the treatment of IAA-producing rhizobacteria isolate Ha21 on wheat cultivar Pasban-90 plants can increase root dry weight by 11.43% when compared to the control.
Rhizobacteria treatment was able to increase the crown dry weight ranging from 53.64% to 128.78% when compared to the control. PAGS treatment increased the crown dry weight by 128.78%, KLPE treatment by 53.64%, KLPN treatment by 55.40%, STMA treatment by 58.51% when
compared to the control. Some researchers also reported similar results that rhizobacteria can increase crown dry weight. Cakmakci et al. (2007) reported that barley seed application treatment with Paenibacillus polymyxa RC05 which produces IAA 32.8 µg/ml in Tryptic Soy Broth (TSB) media with the addition of 25 mg tryptophan increased the crown weight by 54.23% when compared to the control. Ekinci et al. (2014) reported that watering treatment with Bacillus megaterium KBA-10 suspension which produces IAA 6.7 ng/µl in Tryptic Soy Broth increased the dry weight of cauliflower plant crowns (Brassica oleracea L.) by 45.38% when compared to the control.
Rhizobacterial treatment was able to increase leaf chlorophyll levels ranging from 15.37% to 22.55% when compared to the control. PAGS treatment increased leaf chlorophyll content by 22.55%, KLPE treatment by 15.37%, KLPN treatment by 19.09%, STMA treatment by 19.64% when compared to the control. Some researchers also reported similar results that rhizobacteria can increase leaf chlorophyll levels. Mahmood et al. (2016) reported that seed coating treatment of Mung Bean seeds with Bacillus drentensis P16 which produces IAA 3.86 mg/l in Luria Bertani Broth media without the addition of tryptophan increased chlorophyll content by 25.19% when compared to the control. Igbal et al. (2016) reported that seed coating treatment on corn seeds with rhizobacteria isolate MA-11 which produced IAA 12.08 µg/ml on GPM broth media without the addition of tryptophan increased the total chlorophyll content by 13.56% when compared to the control. Yildirim et al. (2015) reported that
eISSN: 2655-9994 pISSN: 2303-3371 https://doi.org/10.24843/IJBB.2022.v10.i01.p04
PGPR treatment in cabbage plants increased leaf chlorophyll by 3.43% when compared to the control. While Turan et al. (2014) reported that treatment of cabbage plant seeds with Bacillus megaterium TV-91C increased leaf chlorophyll by 5.74% when compared to the control. Erdogan et al. (2016) reported that the treatment of Rhodococcus erythropolis RC9 which produced 22.6 µg ml-1 IAA increased leaf chlorophyll of strowberry plants by 28.82% when compared to the control.
The rhizobacterial treatments of P. agglomerans TR78, S. maltophilia KC29 and K. pneumoniae KC66 were able to reduce the root length while K. pneumoniae SW04 was able to increase the root length of chili plants (Figure 3). The rhizobacterial treatments of P. agglomerans TR78, S. maltophilia KC29 and K. pneumoniae KC66 reduced root length by 17.72%, 12.30%, and 8.48% respectively when compared to the control while K. pneumoniae SW04 increased root length by 2.53% when compared to the control. The rhizobacterial treatment was able to reduce and increase the root length presumably due to differences in the concentration of IAA compounds produced by the rhizobacteria. Loper and Schroth (1986) reported the same research results that rhizobacteria are able to reduce root length and increase root length depending on the level of concentration of IAA compounds produced by rhizobacteria, the higher the IAA produced by rhizobacteria, the higher the reduction power of primary root length, where the percentage of reduction power of primary root length of sugar beet plants in the rhizobacterial treatment of P. fluorescens E8 which is high in concentration. fluorescens E8 which
produces IAA 0.57 µg ml-1, rhizobacterial treatment of enterobacteriaceae group isolate 111 which produces IAA 0.79 µg ml-1, rhizobacterial treatment of P. fluorescens B10 which produces IAA 1.22 µg ml-1
respectively by 0.84%, 9.25%, 10.46% while the treatment of P. putida A1 which produces IAA 0.44 µg ml-1 is able to increase root length by 2.88%.
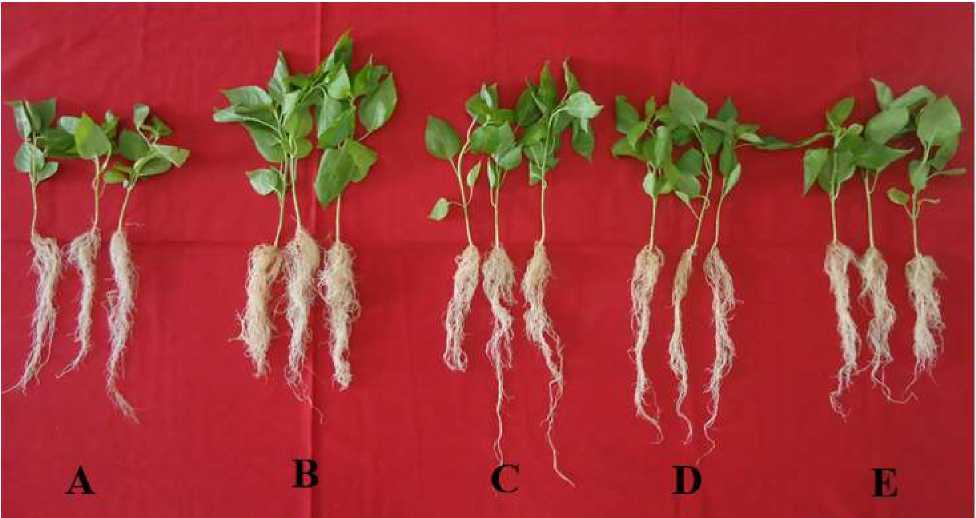
Figure 3. The results of the rhizobacterial ability test in increasing the growth of chili plants A. KLPE treatment; B. PAGS treatment; C. KLPN treatment; D. KTRL treatment; and E. STMA treatment.
Shahzad et al. (2010) reported that the treatment of rhizobacterial isolate JI22 can reduce root length with a reduction power of 8.06% when compared to the control. Kim and Mulkey (1997) reported that IAA in high concentrations will reduce the rate of root elongation, this is because IAA in high concentrations can induce the formation of ethylene and it is known that one of the ways ethylene works is to inhibit the process of elongation of plant cells. Four species of rhizobacteria tested, namely P. agglomerans TR78, K. pneumoniae KC66, K. pneumoniae SW04, and S. maltophilia KC29, have the potential as bioprotectants because they produce substances that are antifungal against the fungus F. oxysporum f.sp. capsici with an antibiosis mechanism and have the potential as biostimulants
because they are able to produce IAA compounds in colorimetric tests using the Salkowski reagent and are able to increase the growth of chili plants.
CONCLUSION
The results of this study concluded the four tested rhizobacterial species have potential as bioprotectants because they are able to inhibit the growth of the fungus F. oxysporum f.sp. capsici with inhibition ranging from 77.33% to 89.79% when compared to the control. Moreover, the four tested rhizobacterial species have potential as biostimulants because they are able to produce IAA compounds in colorimetric tests using Salkowski reagent and are able to increase chili plant growth parameters such as plant height, number of leaves, leaf area,
root dry weight, crown dry weight, leaf chlorophyll content, and root length.
REFERENCES
Asghar, H.N., Zahir, Z.A. Arshad, M. 2004.
Screening rhizobacteria for
improving the growth, yield, and oil content of canola (Brassica napus L.). Australian Journal of Agricultural Research 55: 187 – 194.
Badan Pusat Statistik (BPS). 2022. Produksi cabai rawit di Indonesia tahun 2017 sampai 2021.
https://databoks.katadata.co.id/datapu blish/2022/06/13/pertama-kali-dalam-5-tahun-produksi-cabai-rawit-indonesia-turun-809-pada-2021.
Cakmakci, R., Donmez, M.F., Erdogan, U. 2007. The effect of plant growth promoting rhizobacteria on Barley seedling growth, nutrient uptake, some soil properties, and bacterial counts. Turk. J. Agric. For.31: 189199.
Ekinci, M., Turan, M., Yildirim, E., Gunes, A., Kotan, R., Dursun, A. 2014. Effect of plant growth promoting rhizobacteria on growth, nutrient, organic acid, amino acid and hormone content of Cauliflower (Brassica oleracea L. var. botrytis) transplants. Acta Sci. Pol. Hortorum cultus 13: 71 – 85.
Erdogan, U., Cakmakci, R., Varmazyari, A., Turan, M., Erdogan, Y., Kitir, N. 2016. Role of inoculation with multitrait rhizobacteria on strawberries under water deficit stress. Zemdirbyste Agriculture 103 (1): 67 – 76.
Gusmaini, Aziz, S.A., Munif, A., Sopandie, D., Bermawie, N. 2013. Isolation and selection of endophytic bacteria consortia from medicinal plant (Andrographis paniculata) as plant growth promoting agents. Journal of Agronomy 12 (3): 113 – 121.
Gholami, A., Shahsavani, S., Nezarat, S. 2009. The effect of plant growth promoting rhizobacteria (PGPR) on germination, seedling growth and yield of maize. Word Acad. Sci. Engi. Tech. 49: 19 – 24.
Ismawati. 2016. Strategi manajemen resiko usahatani cabai rawit di Desa Bonto Mate’ne Kecamatan Sinoa
Kabupaten Bantaeng. Program Studi Agribisnis, Fakultas Pertanian, Universitas Muhammadiyah
Makassar.
Igbal, M. A., Khalid, M., Zahir, Z.A., Ahmad, R. 2016. Auxin producing plant growth promoting rhizobacteria improve growth, physiology and yield of maize under saline field conditions. Int. J. Agric. Biol. 18: 37 – 45.
Kamnev, A.A., Shchelochkov, A.G., Tarantilis, P.A., Polissiou, M.G., Perfiliev, Y.D. 2001. Complexation of indole-3-acetic acid with iron(III): Influence of coordination on the π-electronic system of the Ligand. Monatshefte fur Chemie 132: 675 – 681.
Khalid, A., Arshad, M., Zahir, Z.A. 2004. Screening plant growth promoting rhizobacteria for improving growth and yield of wheat. Journal of Applied Microbiology 96: 473 – 480.
Loper, J.E., Schroth, M.N. 1986. Influence of bacterial sources of indole-3-acetic acid on root elongation of sugar beet. Physiology and Biochemistry 7 (4): 386 – 389.
Mayadianti, I.A. I., Khalimi, K., Suniti, W. 2020. Uji Daya Hambat Bakteri Paenibacillus Polymyxa Terhadap Pertumbuhan Jamur Colletotrichum sp. Secara In Vitro. Jurnal Agroekoteknologi Tropika 9(4): 229-237.
Mahmood, S., Daur, I., Solaimani, S.A., Ahmad, S., Madkour, M.H., Yasir, M., Hirt, H., Ali, S., Ali, Z. 2016. Plant growth promoting rhizobacteria and silicon synergistically enhance salinity tolerance of Mung Bean. Front. Plant Sci. 7: 1 -14.
Meudt, W.J., Gaines, T.P. 1967. Studies on the Oxidation of Indole-3-acetic acid by peroxidase enzymes. I. Colorimetric determination of Indole-3-acetic acid Oxidation products. Plant Physiol. 42: 1395 – 1399.
Park, Y.S., Park, K., Kloepper, J.W., Ryu, C.M. 2015. Plant growth promoting rhizobacteria stimulate vegetative growth and asexual reproduction of Kalanchoe daigremontiana. Plant Pathol. J., 3 (3): 310 – 315.
Qi, J., Aiuchi, D., Tani, M., Asano, S. and Koike, M. 2016. Potential of Entomophatogenic Bacillus
thuringiensis as Plant Growth Promoting Rhizobacteria and Biological Control Agents for
Tomato Fusarium Wilt. J. IJOEAR, 2: 55-63.
Rahman, A., Sitepu, I.R., Yan T.S., Hashidoko, Y. 2010. Salkowski’s reagent test as a primary screening index for functionalities of Rhizobacteria isolated from wild
dipterocarp saplings growing
naturally on medium strongly acidic tropical peat soil. Biosci. Biotechnol. Biochem. 74 (11): 1 - 7.
Rajan, A., Radhakrishna, D. 2013. Effect of entophytic bacteria on the rooting and establishment of Hibiscus rosasinensis. IOSR Journal of Agriculture and Veterinary Science 3 (2): 17 – 21.
Rathaur, P., Raja, W., Ramteke, P.W., John, S.A. 2012. Effect of UV-B tolerant plant growth promoting rhizobacteria (PGPR) on seed germination and growth of Withania somnifera. Advances in Applied Science Research 3 (3): 1399 – 1404.
Shahzad, S.M., Khalid, A., Arshad, M., Rehman, K.U. 2010. Screening rhizobacteria containing ACC-deaminase for growth promotion of chickpea seedlings under axenic conditions. Soil and Environ. 29 (1): 38 – 46.
Sivasankari, B., Anandharaj, M. 20014b. Isolation and molecular
characterization of potential plant growth promoting Bacillus cereus GGBSTD1 and Pseudomonas spp. GGBSTD3 from vermisources. Advances in Agriculture 2014: 1 – 13.
Sudharani, M., Shivaprakash, M.K., Prabhavathi, M.K. 2014. Role of consortia of biocontrol agents and PGPR’s in production of cabbage under nursery condition.
Int.J.Curr.Microbiol.App.Sci 3(6): 1055-1064.
Swain, M.R., Naskar, S.K., Ray, R.C. 2007.
Indole-3-acetic acid production and
effect on Sprouting of Yam (Dioscorea rotundata L.) minisetts by Bacillus subtilis isolated from Culturable Cowdung Microflora. Polish Journal of Microbiology 56 (2): 103 – 110.
Turan, M., Ekinci, M., Yildirim, E., Gunes, A., Karagoz, K., Kotan, R., Dursun, A. 2014. Plant growth promoting rhizobacteria improved growth, nutrient, and hormone content of cabbage (Brassica oleracea)
seedlings. Turkish Journal of Agriculture Forestry 38: 327 – 333.
Wahyudi, A.T., Astuti, R.P., Widyawati, A., Meryandini, A., Nawangsih, A.A. 2011. Characterization of Bacillus sp. strain isolatd from rhizosphere of soybeanR plants for their use as potential plant growth for promoting rhizobacteria. Journal of
Microbiology and Antimicrobials 3 (2): 34 - 40.
Yaqoob, A., Farooq, N., Sajid, I., Ali, B. 2013. Auxin production by Azospirillum: Role in growth
promotion of Triticum aestivum L. and Lens culinaris Medik. Global Journal of Scientific Researches 1 (1): 26 – 32.
Yildirim, E., Turan, M., Ekinci, M., Dursun, A., Gunes, A., Donmez, M.D. 2015a. Growth and mineral content of cabbage seedlings in response to nitrogen fixing rhizobacteria treatment. Romanian
Biotechnological Letters 20 (6):
10929 – 10935.
Zahid, M., Abbasi, M.K., Hameed, S., Rahim, N. 2015. Isolation and identification of indigenous plant growth promoting rhizobacteria from Himalayan region of Kashmir and their effect on improving growth and nutrient contents of maize (Zea mays L.). Frontiers in Microbiology 6: 1 – 10.
36
Discussion and feedback