NON-HOST RESISTANCE ACTIVITIES OF Arabidopsis thaliana INDUCED BY METHANOL EXTRACT OF MYCELIA FROM Phytophthora infestans
on
ARTICLE
NON-HOST RESISTANCE ACTIVITIES OF Arabidopsis thaliana INDUCED BY METHANOL EXTRACT OF MYCELIA FROM Phytophthora infestans
Mohammad Shahjahan Monjil, Shinya Wada, Daigo Takemoto and Kazuhito Kawakita*
Plant Pathology Laboratory, Graduate School of Bioagricultural Sciences, Nagoya University, Chikusa, Nagoya, 464-8601, Japan *Corresponding author: [email protected]
ABSTRACT
Defense system of a non-host pathosystem involving the model plant Arabidopsis thaliana and methanol extract of mycelia (MEM) elicitor from Phytophthora infestans are presented in this article. A. thaliana leaves were analyzed for the induction of reactive oxygen species (ROS), hypersensitive reaction (HR) like cell death as well as defense gene expression by MEM elicitor. MEM elicitor induced O2¯ generation in A. thaliana leaves with dose dependent nature. HR occurred during the elicitation of A. thaliana challenged with MEM elicitor. Responses of intact A thaliana seedlings to MEM elicitor caused the retardation of growth of seedlings. Both plant biomass and growth of primary roots was markedly reduced by the treatment of MEM. MEM elicitor induced the expression of defense genes such as pathogenesis related proteins, PR1, PR2, PR4 and PR5, and rbohF encoding a ROS generation. These results suggested that MEM elicitor induced non-host defense responses in A. thaliana.
Keywords: methanol extract of mycelia, defense activities, Phytophthora infestans, Arabidopsis thaliana, elicitor
Abbreviations:
DMSO, Dimethyl sulfoxide; HWC, Hyphal wall components; L-012, 8-Amino-5-chloro-7-phenylpyridol[3,4-d] pyridazine-1,4(2H,3H) dione sodium salt; MES, 2-(N-morpholino)ethanesulfuric acid; MOPS, 3-(N-morpholino)propanesulphonic acid; MS medium, Murashige-Skoog basal medium; HR, Hypersensitive response; PCD, Programmed cell death; Rboh, Respiratory burst oxidase homolog; ROS, Reactive oxygen species.
INTRODUCTION
Plants are challenged by numerous pathogens throughout their life cycles and yet are able to fend off most infections. The native resistance of most plant species against a wide variety of pathogens is known as nonhost resistance (NHR), which confers durable protection to plant species. Recognition of nonself is the key to the activation of innate defense mechanisms in plants in response to microbial attacks. The innate defense system depends on the detection of microbial elicitors that induces a cascade of defense responses that may include MAP kinase activity, the up-regulation of antimicrobial compounds, elicitation of inflammatory responses, the production of cytokines or mucins, apoptosis, nitric oxidemediated responses, and the transcription of defense related genes (Chisholm et al, 2006;
Medzhitov, 2007; Schwessinger and Zipfel, 2008).
The most notorious oomycetes are Phytophthora species, arguably the most devastating pathogens of dicot plants (Kamoun, 2003). Phytophthora infestans causes late blight, a devastating disease that results in multibilliondollar losses in potato and tomato production (Garelik, 2002; Smart and Fry, 2001). Most plants, such as weeds and various crops, are resistant to P. infestans, and grow unimpaired in or next to fields with a high incidence of late blight (Kamoun et al., 1999). Understanding the molecular basis of non-host resistance to P. infestans will provide insight into a key molecular process and will offer novel perspectives for engineering durable late blight resistance in crop plants.
Species-specific elicitors have been described in P. infestans and other Phytophthora species and can trigger defense responses in non-
host plants. An extracellular transglutaminase that is conserved in P. infestans and other Phytophthora species induces defense responses in the non-host parsley (Brunner et al., 2002; Nurnberger and Brunner, 2002). Arabidopsis thaliana has emerged as a valuable model to study NHR in plants (Holub and Cooper, 2004; Yun et al., 2003). A series of penetration mutants were identified in the model plant A. thaliana, allowing considerable progress towards understanding NHR. Huitema et al. (2003) reported that active defense responses associated with non-host resistance of A. thaliana to the oomycete pathogen P. infestans.
Several plant defense responses are induced during the NHR response. Many defense responses are similar to those induced during gene-for-gene or host resistance. A rapid and transient production of reactive oxygen species (ROS), termed 'oxidative burst' is a hallmark of successful recognition of plant pathogens and the oxidative burst could have a direct effect on pathogen or the defenses caused in plant because of its reactivity (Torres, 2010). ROS are produced during both gene-for gene and NHR, even though the timing and amount might slightly vary between the two (Huckelhoven, 2001, Able et al., 2003).
ROS also associated with the hypersensitive reaction (HR), a localized response at the site of pathogen attack that displays programmed cell death and that could contribute to limit the spread of the pathogens or be a source of signals for establishment of further defenses (Mur et al., 2008). HR is associated with both host and nonhost resistance to P. infestans (Vleeshouwers, et al., 2000). HR occurs at the infection site with apoptosis-like features. Despite controversial debate as to the role of ROS in the plant HR, they are generally regarded as causes of hypersensitive cell death (Delledonne et al., 2001, Torres et al., 2005).
In this study, we used methanol extract of mycelia (MEM) from P. infestans for the study of induced resistant reactions in non-host model plant A. thaliana.
MATERIALS AND METHODS
Plant materials
A. thaliana ecotype Columbia-0 (Col-0) plants were grown at 23°C and 70% humidity under a 16 h photoperiod and a 8 h dark period in environmentally controlled growth cabinets and 3-4 weeks old leaves were used for the experiments. For the study of plant growth, seeds were surface sterilized with 1% sodium hypochlorite, rinsed in water, and placed at 4°C for 3-4 days in darkness. Seeds were then plated on Murashige and Skoog (MS) media supplemented with 3% sucrose and solidified with 0.1% Phytagel. MEM elicitor was dissolved in water by adding 3% DMSO and was added separately into the medium and mixed well before gelation. Seeds were plated on medium containing MEM elicitor (or control medium) and effects assayed 6 days later.
Pathogenic isolate Phytophthora infestans
The pathogenic isolate [Phytophthora infestans (Mont.) De Bary], race 1.2.3.4 was used to culture for mycelia collection and were maintained at Plant Pathology laboratory of the Graduate School of Bioagricultural Sciences, Nagoya University. The fungi used as inocula for liquid culture were grown on rye-seed extract agar medium in a test tube at 18°C in the dark condition for 2 weeks. Parts of the growing mycelia were placed in 100 ml Erlenmeyer flasks containing 20 ml of rye liquid nutrient medium of rye seed-extract (60 g rye seed), 20 g of sucrose and 2 g of yeast extract per 1 liter of distilled water and incubated in dark condition for 2 weeks at 18°C to allow growth of the mycelia. The mycelial mats grown in the liquid medium were washed thoroughly with running tap water on a mesh (3 × 3 mm) to remove the growth medium and zoosporangia and finally washed with distilled water. To remove excess water, water was filtered through filter paper (Toyoroshi No. 2) under reduced pressure and then frozen at -20°C for preparation of MEM elicitor.
Preparation of MEM elicitor from Phytophthora infestans
The collected mycelia of P. infestans were frozen with liquid nitrogen. The frozen mycelia were ground by a mortar and pestle under a freezing temperature using liquid nitrogen. The ground mycelia were transferred to falcon tube (50 ml) containing methanol at the rate of 10 ml of methanol per 1 g mycelia. The mycelia suspension were finely ground using a polytron type homogenizer (HG30, Hitachi Koki, Japan) for 2 min. After centrifugation at 4°C, 3000 × g for 30 min, the supernatant was collected and dried by using an evaporator, and was used as MEM elicitor.
Measurement of ROS production
The relative intensity ofROS generationwas measured bycountingphotonsfrom L-012 (Waku, Osaka, Japan)-mediated chemiluminescence. In A. thaliana leaves, ROS measurement was done as described by Kobayashi et al. (2007) with little modification. For the detection of ROS production in leaf tissues, 0.5 mM L-012 in 10 mM MOPS-KOH (pH 7.4) was used to infiltrate in Arabidopsis leaves via a syringe without needle. Chemiluminescencewasmonitoredcontinuously using a photon image processor equipped with a sensitive CCD camera in the dark chamber at 20°C (Aquacosmos 2.5; Hamamatsu Photonics, Shizuoka, Japan) and quantified using the U7501 program (Hamamatsu Photonics).
Detection of cell death
To detect cell death on the plant, elicitors were infiltrated by using a syringe without needle from opposite surface of the leaves. Cell death was measured by an electrolyte leakage method, which was adapted from the method described by Yeom et al. (2011). Leaves were infiltrated by elicitors under the leaf surface, and leaf discs (1 cm in diameter) were collected from the leaf 12, 24 and 48 h after treatment. Seven leaf discs were floated on 7 ml of distilled water for 2 h at room temperature and electrical conductivity was measured using a conductivity meter (Horiba, Kyoto, Japan).
Microscopic observation of cell death
To visualize plant cell death and colonization of P. infestans, leaves of A. thaliana were stained with lactophenol trypan blue as described by Takemoto et al. (2003) with a minor modification. Briefly, infected leaves were cleared in methanol overnight, and then the cleared tissue was boiled for 2 min in lactophenol trypan blue stain (10 ml H2O, 10 ml lactic acid, 10 ml glycerol, 10 g phenol, and 10 mg trypan blue). After the leaves had been allowed to cool at room temperature for 1 h, the stain was replaced with 1 g/m1 chloral hydrate. Stained leaves were monitored using an Olympus microscope BX51 (Olympus).
RNA preparation and RT-PCR
Total RNAs were isolated from MEM
treated frozen Arabidopsis leaves using TRIzol reagent (Invitrogen, Carlsbad, CA, USA) and reverse-transcribed as previously described (Kato et al., 2008). Expression of Arabidopsis defense related-genes after the treatment of elicitors was analyzed by RT-PCR. RT-PCR was conducted using a commercial kit (ReverTra-Plus-; Toyobo, Osaka, Japan). The cDNA was synthesized from total RNA (1 μg) with an oligo (dT) primer. After the cDNA synthesis reaction, the PCR was performed with denaturing, annealing, and extension temperatures of 94°C for 15 s, 55°C for 30 s, and 72°C for 30 s, respectively. Gene-specific primers for each sequence were as follows:
GTGGTGCTAAGAAGAGGAAGA-3′,
TCAAGCTTCAACTCCTTCTTT-3′,
GTAGGTGCTCTTGTTCTTCCC-3′,
CACATAATTCCCACGAGGATC-3′,
CTACAGAGATGGTGTCA-3′, AtPR2 reverse primer, 5′-AGCTGAAGTAAGGGTAG-3′,
AACAACCCTGACCTTGTTGC-3′, AtPR3 reverse primer,5′-
ACGTCCACACTCCAATCCAC-3′, AtPR4 forward primer, 5′-CTTGTCCCGGTAACATCTGC-3′, AtPR4 reverse primer, 5′-TGGAGCAATAAGCACTCACG-3′, AtPR5 forward primer, 5′-CACATTCTCTTCCTCGTGTTC-3′, AtPR5 reverse primer, 5′-TAGTTAGCTCCGGTACAAGTG-3′, AtrbohD forward primer, 5′-CATGAACTTGGGATTCTACGAGG-3′, AtrbohD reverse primer, 5′-AGACCTTTGAGTGCGTGGATGG-3′, and AtrbohF forward primer, 5′-GACGATGACACAATCGTTCTTCGT-3′, AtrbohF reverse primer, 5′-TTGAGCGAAATCGGAGCGATAGAT-3′.
RESULTS AND DISCUSSION
O2¯ producing activity
MEM elicitor contains relatively low molecular weight compounds because of limited solubility of high molecular weight compounds or protein in methanol. The oxidative burst (rapid and transient production of O2¯) in A. thaliana plant was detected after MEM elicitor treatment. MEM was infiltrated into A. thaliana leaves at the concentration of 0, 0.25, 0.5, 0.75 or 1 mg/ml and incubated for 24 h. O2¯ producing activity was measured by using O2¯ unique luminous reagent L-012 (Fig. 1). MEM is a high O2¯ producing elicitor with dose dependent nature (Fig. 1A and B). Three % DMSO was used as a negative control because MEM elicitor was dissolved in 3% DMSO. Thus, MEM elicitor induced O2¯ generation in non-host A. thaliana as an early response and recognition of elicitor by plant.
Induction of cell death
HR-like cell death was examined in A. thaliana leaves. Ion leakage has been observed as an indicator of plant cell death (Rizhsky et al., 2002). In this experiment, average-sized A. thaliana leaves (21 days after germination) were selected and infiltrated by MEM elicitors by using
syringe without needle from lower surface of the leaves. Leaf discs collected from treated leaves were floated on distilled water and shaken for 2 h at room temperature. Higher degree of ion leakage was detected in MEM elicitor-treated leaves after 12, 24 and 48 h compared to DMSO-treated leaves (Fig. 2A). The interaction between MEM elicitor and A. thaliana was examined using light microscopy after staining with lactophenol trypan blue of inoculated leaves because staining of plant cells with trypan blue is indicative of cell death. The densely stained epidermal and mesophyll cells were observed in the MEM treated leaves from 3 days after inoculation (Fig. 2B). On the other hand, no stained areas were developed in DMSO treated A. thaliana leaves. These results confirm that MEM causes cell death in non-host A. thaliana plant.
Root growth is arrested in response to MEM elicitor
To study the effects of MEM elicitor on root growth, we germinated seeds in the presence of the MEM elicitor and examined the effects on the roots after 6 days. Inhibition of growth of the A. thaliana seedlings treated with MEM elicitor was observed (Fig. 3A). Retardation of plant growth was apparent irrespective to seedling biomass and root growth (Fig. 3B and C). Thus, it was indicated that the treatment with MEM elicitor sensitized the plant which causes internal changes, and consequently root growth was markedly retarded.
Expression of defense-related genes
To investigate whether the defense-related genes are induced by MEM elicitor, total RNAs from MEM-treated A. thaliana leaves were extracted and were analyzed by RT-PCR (Fig. 4). We checked salicylic acid associated defense gene PR (pathogenesis-related protein) namely AtPR1, AtPR2, AtPR3, AtPR4 and AtPR5. High expression of AtPR1, AtPR2, AtPR4 and AtPR5 genes was found by MEM elicitor treatment. MEM elicitor also induced ROS production regulatory genes, Rbohs (respiratory burst
oxidase homologue). Expression of StrbohF gene was observed in A. thaliana. Therefore, MEM-induced gene expression of AtrbohF and PR gene series, AtPR1, AtPR2, AtPR4 and AtPR5, are involved in non-host defense response in A. thaliana.
One of the most rapid defense reactions to pathogen attack is the so-called oxidative burst, which constitutes the production of O2¯ at the site of attempted invasion (Apostol et al., 1989). In the present study, it was shown that MEM elicitor from P. infestans induced O2¯ production in non-host A. thaliana (Fig. 1). The involvement of ROS in non-host response has been found in various plant-pathogen systems (Wen et al., 2011, Hückelhoven and Kogel, 2003, Nimchuk et al., 2003). ROS are produced during both gene-for gene and nonhost resistance, even though the timing and amount might slightly vary between the two (Huckelhoven, 2001, Able et al., 2003). These results, in conjunction with the present
study, provide evidences that MEM elicitor from P. infestans induced ROS in non-host A. thaliana-elicitor or -pathogen system.
An elicitor can be recognized by the plant surveillance system and a defense reaction leading to HR will be activated (Mysore and Ryu, 2004). MEM elicitor induced HR in nonhost A. thaliana (Fig. 2). Chloroplast-generated ROS play a major role in localized cell death during the non-host interaction between tobacco and Xanthomonas campestris pv. vesicatoria (Zurbriggen et al., 2009). Several lines of evidence from various plant species suggest that the sources of ROS are different during non-host response and during the HR, but these sources may interact with each other (Bindschedler et al., 2006, Soylu et al., 2005, Torres et al., 2005). These results indicate that both host and non-host specific interactions can provide similar kind of HR response even though the reduced degree of response appears in non-host interaction.
B
A 0 0.25 0.5 0.75 1.0 (mg/ml)
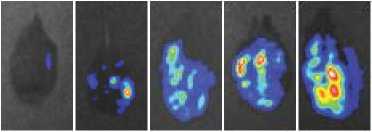
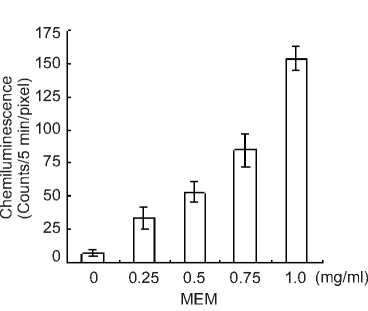
Fig. 1. O2- inducing activity of MEM in A. thaliana leaves. A, Leaves of A. thaliana ecotypes (Col-0) were treated with 0, 0.25, 0.5, 0.75 or 1.0 mg/ml MEM elicitor in 3% DMSO. Production of O2- was detected 24 h after treatment as L-012 mediated chemiluminescence. Chemiluminescence images shown were obtained using CCD camera. B, Intensities of chemiluminescence were quantified with photon image processor. Data are means standard deviation from three independent experiments.
B
A
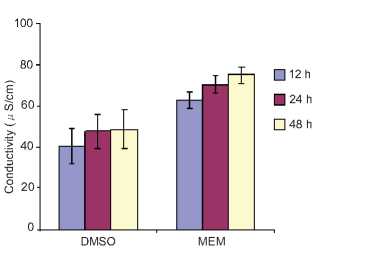
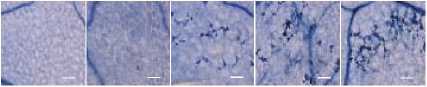
0 0.25 0.5 0.75
MEM
1.0 (mg/ml)
Fig. 2. Induction of cell death by MEM in A. thaliana leaves. A, Electrolyte leakage of dead cells in A. thaliana leaves. Leaves were infiltrated with 1.0 mg/ml of MEM and incubated for 12, 24 and 48 h. After treatment, leaf discs were floated on distilled water and shaken for 2 h at room temperature. Electrical conductivity was measured using a conductivity meter. Data are means standard deviation from five replications. B, Microscopic observation of hypersensitive cell death induced by MEM elicitor in A. thaliana ecotype (Col-0). Leaves of A. thaliana were treated with 0 l, 0.25, 0.5, 0.75 or 1.0 mg/ml MEM. Hypersensitive cell death was detected by trypan blue staining at 3 days after treatment. Bars = 200 mm
Root growth is highly responsive to environmental signals. To access the response of intact and growing plants to MEM elicitor, we examined the morphogenic changes in A. thaliana seedlings treated with the elicitor. Our results demonstrated that MEM induced the growth retardation (Fig. 3) concomitantly with the defense responses in A. thaliana seedlings. Kawaguchi et al. (2011) reported that fungal elicitor-induced retardation and its restoration of root growth in tobacco seedlings.
An incompatible interaction of a pathogen and a nonhost plant often induces several different defense signaling cascades, including generation of ROS, programmed cell death or HR in infected cells, and induction of PR genes. We examined the expression profiles of typical genes involved in various defense and metabolic pathways. Elicitor induced defense genes such as pathogenesis related proteins (PR-1, PR-2, PR-5) involved in systemic acquired resistance (Rogers and Ausubel, 1997), and two homologues of the human respiratory burst oxidase (gp91phox)
such as rbohD and rbohF (Torres et al., 1998). In our present study, well studied defense genes AtPR1, AtPR2, AtPR4 and AtPR5, and AtrbohF encoding ROS generation were induced by MEM elicitor regardless of the ROS levels in A. thaliana leaves. Thus, these results suggested that MEM elicitor induced non-host defense responses are activated in A. thaliana.
MEM
A
MEM +
MEM
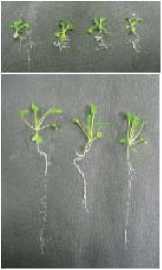
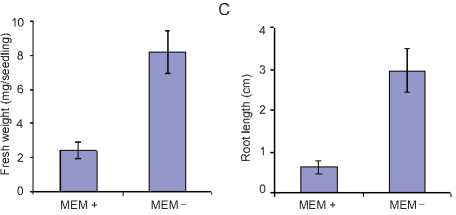
Fig. 3. Effect of MEM elicitor on growth of A. thaliana ecotype Col-0. A, A. thaliana were grown on MS media supplemented with or without 1.0 mg/ml MEM elicitor for 2 weeks. B, Fresh weight of Arabidopsis seedlings in response to 1.0 mg/ml MEM. Data are means SE from at least 10 seedlings. C, Root growth in response to 1.0 mg/ml MEM. Data are means SE from at least 10 seedlings.
Fig. 4. Gene expression in MEM treated A. thaliana leaves. Total RNAs were isolated from A. thaliana leaves treated with MEM at concentration of 100 g/m for 24 h. Three % DMSO was used as a control. RNAs were analyzed by RT-PCR using specific primers for AtPR1, AtPR2, AtPR3, AtPR4, AtPR5, AtrbohD, and AtrbohF. Equal loads of cDNA were monitored by amplification of constitutively expressed AtUBQ5.
ACKNOWLEDGEMENTS
This research was supported by Adaptable and Seamless Technology Transfer Program through Target-driven R&D (AS221Z03302E) from Japan Science and Technology Agency.
REFERENCES
Able AJ, Sutherland MW and Guest DI. 2003.
Production of reactive oxygen species during non-specific elicitation, non-host resistance and field resistance expression in cultured tobacco cells. Funct. Plant Biol. 30: 91–99.
Apostol I, Heinstein PF and Low PS. 1989. Rapid stimulation of an oxidative burst during elicidation of cultured plant cells. Role in defense and signal transduction. Plant Physiol. 90: 106–116.
Bindschedler LV, Dewdney J, Blee KA, Stone JM, Asai T, Plotnikov J, Denoux C, Hayes T, Gerrish C, Davies DR, Ausubel FM and Bolwell GP. 2006. Peroxidase-dependent apoplastic oxidative burst in Arabidopsis required for pathogen resistance. Plant J. 47: 851–863.
Brunner F, Rosahl S, Lee J, Rudd JJ, Geiler C, Kauppinen S, Rasmussen G, Scheel D and Nurnberger T. 2002. Pep-13, a plant defenseinducing pathogen associated pattern from Phytophthora transglutaminases. EMBO J. 16: 6681–6688.
Chisholm ST, Coaker G, Day B and Staskawicz BJ. 2006. Host-microbe interactions: Shaping the evolution of the plant immune response. Cell 124: 803–814.
Delledonne M, Zeier J, Marocco A and Lamb C. 2001. Signal interactions between nitric oxide and reactive oxygen intermediates in the plant hypersensitive disease resistance response. Proc. Natl. Acad. Sci. USA 98: 13454–13459.
Garelik G. 2002. Agriculture. Taking the bite out of potato blight. Science 298: 1702–1704.
Holub EB and CooperA. 2004. Matrix, reinvention in plants: How genetics is unveiling secrets of non-host disease resistance. Trends Plant Sci. 9: 211-214.
Hückelhoven R, Dechert C and Kogel KH. 2001. Non-host resistance of barley is associated with a hydrogen peroxide burst at sites of attempted penetration by wheat powdery mildew fungus. Mol. Plant Pathol. 2: 199205.
Hückelhoven R and Kogel KH. 2003. Reactive oxygen intermediates in plant-microbe interactions: who is who in powdery mildew resistance? Planta 216: 891–902.
Huitema E, Vleeshouwers VGAA, Francis DM and Kamoun S. 2003. Active defence responses associated with non-host
resistance of Arabidopsis thaliana to the oomycete pathogen Phytophthora infestans. Mol. Plant Pathol. 4: 487-500.
Kamoun S. 2003. Molecular genetics of pathogenic oomycetes. Euk. Cell 2: 191–199.
Kamoun S, Huitema E and Vleeshouwers VGAA. 1999. Resistance to oomycetes: a general role for the hypersensitive response? Trends Plant Sci. 4: 196–200.
Kato H, Asai S, Yamamoto-Katou A, Yoshioka H, Doke N and Kawakita K. 2008. Involvement of NbNOA1 in NO production and defense responses in INF1-treated Nicotiana benthamiana. J. Gen. Plant Pathol. 74: 15– 23.
Kobayashi M, Ohura I, Kawakita K, Yokota N, Fujiwara M, Shimamoto K, Doke N and Yoshioka H. 2007. Calcium-dependent protein kinases regulate the production of reactive oxygen species by potato NADPH oxidase. Plant Cell 19: 1065–1080.
Medzhitov R. 2007. Recognition of microorganisms and activation of the immune response. Nature 449: 819–826.
Mur LA, Kenton P, Lloyd AJ, Ougham H and Prats E. 2008. The hypersensitive response; the centenary is upon us but how much do we know? J . Exp. Bot. 59: 501–520.
Mysore KS and Ryu C-M. 2004. Nonhost resistance: how much do we know? Trends Plant Sci. 9: 97–104.
Nimchuk Z, Eulgem T, Holt BF and Dangl JF. 2003. Recognition and response in the plant immune system. Annu. Rev. Genet. 37: 579–609.
Nurnberger T and Brunner F. 2002. Innate immunity in plants and animals: emerging parallels between the recognition of general elicitors and pathogen-associated molecular patterns. Curr. Opin. Plant Biol. 5: 318–324.
Rizhsky L, Liang H and Mittler R. 2002. The combined effect of drought stress and heat shock on gene expression in tobacco. Plant Physiol. 130: 1143–1151.
Rogers EE and Ausubel FM. 1997. Arabidopsis enhanced disease susceptibility mutants
exhibit enhanced susceptibility to several bacterial pathogens and alterations in PR-1 gene expression. Plant Cell 9: 305–316.
Schwessinger B and Zipfel C. 2008. News from the frontline: Recent insights into PAMP-triggered immunity in plants. Curr. Opin. Plant Biol. 11: 389–395.
Smart CD and Fry WE. 2001. Invasions by the late blight pathogen: renewed sex and enhanced fitness. Biol. Invas. 3: 235–243.
Soylu S, Brown I and Mansfied JW. 2005. Cellular reactions in Arabidopsis following challenge by strains of Pseudomonas syringae: from basal resistance to compatibility. Physiol. Mol. Plant Pathol. 66: 232–243.
Torres MA, Jones JD and Dangl JL. 2005. Pathogen-induced, NADPH oxidasederived reactive oxygen intermediates suppress spread of cell death in Arabidopsis thaliana. Nature Genet. 37: 1130–1134.
Torres MA, Onouchi H, Hamada S, Machida C, Hammond-Kosack KE and Jones JD. 1998. Six Arabidopsis thaliana homologues of the human respiratory burst oxidase (gp91phox). Plant J. 14: 365–370.
Torres M. 2010. ROS in biotic interactions. Physiol. Plantarum 138: 414–429.
Takemoto D, Jones DA and Hardham AR. 2003. GFP-tagging of cell components reveals the dynamics of subcellular re-organization in response to infection of Arabidopsis by oomycete pathogens. Plant J. 33: 775–792.
Vleeshouwers VGAA, van Dooijeweert W, Govers F, Kamoun S and Colon LT. 2000. The hypersensitive response is associated with host and nonhost resistance to Phytophthora infestans. Planta 210: 853–864.
Wen Y, Wang W, Feng J, Luo MC, Tsuda K, Katagiri F, Bauchan G and Xiao S. 2011. Identification and utilization of a sow thistle powdery mildew as a poorly adapted pathogen to dissect post-invasion nonhost resistance mechanisms in Arabidopsis. J. Exp. Botany 62: 2117–2129.
Kawaguchi Y, Nishiuchi T, Kodama H, Nakano T, Nishimura K, Shimamura K, Yamaguchi K, Kuchitsu K, Shinshi H and Suzuki K. 2012. Fungal elicitor-induced retardation and its restoration of root growth in tobacco seedlings. Plant Growth Regul. 66: 59-68.
Yeom SI, Baek HK, Oh SK, Kang WH, Lee SJ, Lee JM, Seo E, Rose JKC, Kim BD and Choi D. 2011. Use of a secretion trap screen in pepper following Phytophthora capsici infection reveals novel functions of secreted plant proteins in modulating cell death. Mol. Plant-Microbe Interact. 24: 671–684.
Yun BW, Atkinson HA, Gaborit C, Greenland A, Read ND, Pallas JA and Loake GJ. 2003. Loss of actin cytoskeletal function and EDS1 activity, in combination, severely compromises non-host resistance in Arabidopsis against wheat powdery mildew. Plant J. 34: 768-777.
Zurbriggen MD, Carrillo N, Tognetti VB, Melzer M, Peisker M, Hause B and Hajirezaei M-R. 2009. Chloroplast-generated reactive oxygen species play a major role in localized cell death during the nonhost interaction between tobacco and Xanthomonas campestris pv. vesicatoria. Plant J. 60: 962–973.
udayana university biosciences and biotechnology forum • 85
Discussion and feedback