DEVELOPMENT OF IN PLANTA TRANSFORMATION METHOD USING Agrobacterium tumefaciens THAT IS SIMPLE AND EFFICIENT AS WELL AS APPLICABLE TO VARIOUS PLANTS
on
ARTICLE
Review
DEVELOPMENT OF IN PLANTA TRANSFORMATION METHOD USING Agrobacterium tumefaciens
THAT IS SIMPLE AND EFFICIENT AS WELL AS APPLICABLE
TO VARIOUS PLANTS 1)
Mineo Kojima
Professor Emeritus, Shinshu University, Japan2-46, Gokuraku, Meito-ku, Nagoya, 465-0053 Japan Phone & Fax: +81-(0)52-701-3873 Email: kojima.m@silk.plala.or.jp
1) This is a revised paper with addition of some new data of: Kojima, M., Suparthana. P., and Nogawa, M.; Journal of Japanese Science of Bioengineering
(Seibutukogakukaishi) 85, 57-62 (2007)
ABSTRACT
A simple and efficient in planta transformation method was developed. In the method, meristems of either apical or axillary buds of immature plants or apical buds of embryos in imbibed seeds, depending on plants, were inoculated by Agrobacterium tumefaciens after being pricked with a needle. The inoculated plants were grown to maturation in pots under non-sterile conditions. Transformation was demonstrated by several lines of evidence obtained with mostly the progenies of T1 generation; phenotypic inheritance from T0 plants to plants of the following generation, resistance of seed germination to antibiotics, detection of β-glucuronidase activity in transformants of T1 generation, detection of transgene by Southern blot and PCR analyses in T1 generation transformants and rescue from T1 generation transformants of the plasmids composed of T-DNA of binary vector and flanking plant genomic DNA. The diverse species of plants such as buckwheat (Fagopyrum esculentum), mulberry (Morus alba L.), kenaf (Hibiscus cannabinus), rice (Oryza sativa), wheat (Triticum aestibvum L.), maize (Zea mays), and soybean (Glycine max) were shown to be efficiently transformed by our in planta method.
Keywords: in planta transformation, Agrobacterium tumefaciens
Conventional transformation methods and
their disadvantages
In the conventional transformation methods being currently used, transgene is first introduced into either calli or tissues
in vitro culture, using either Agrobacterium-mediated method or microparticle bombardment method and then plants are regenerated on medium. The Agrobacteium-mediated method has several advantages over the microparticle bombardment method: the former allows for a more stable integration of a defined segment of DNA into plant genome and generally results in a lower copy number and fewer rearrangements than the latter.
Although the conventional transformation methods are commonly used, they have some disadvantages. First, they require sterile
conditions. Second, they take rather long periods of time. Third, somatic mutation or somaclonal variation occurs frequently in plant cells during in vitro culture. Finally, some plants are recalcitrant to regeneration. These disadvantages of the conventional methods are mainly caused by in vitro culture of plant cells. Therefore, some in planta transformation methods, which require no in vitro culture of plant cells, have been reported to overcome the disadvantages of the conventional methods (Chee et al., 1989; Touraev et al.1997; Clough et al., 1998; Hu et al., 1999; Qing et al., 2000). However, none of the reported in planta transformation methods except one for Arabidopsis have been widely used because of the problem of efficiency and reproducibility (Bent, 2000). On the other hand, the in planta transformation method, or
floral dip method, for Arabidopsis is reliable and thereby widely used. Unfortunately, the method is, however, applicable to only Arabidopsis. Thus, we attempted to develop the general in planta transformation method that can be applied to various plants.
The hint for development of new method
In our method, a meristem was selected as a target tissue for inoculation of Agrobacterium. The hint for this idea came from the experiment as follows. Kalanchoe pinnata has many adventitious buds on the edge of its leaf (Fig. 1A). Each bud contains a meristem with totipotency and thereby is capable to produce a whole plant. When a leaf is detached from stem and kept on soil or wet vermiculite, a young plantlet emerges from each bud in two or three weeks. The leaves from one plant are a clone, having an identical genome.
The adventitious buds of one leaf were inoculated, after being pricked with a needle, by
Fig. 1. Adventitious buds on leaf blade of Kalanchoe pinnata Lam (A) and appearance of the plants emerged from the adventitious buds inoculated by A.tumefaciens (B). The plants of B- (1) are ones emerged from the buds inoculated by A. tumefaciens (LBA4404) strain containing a 2.5 kb of buckwheat cDNA with unknown function in a sense orientation. The plants of B- (2) are ones emerged from the buds inoculated by A.tumefaciens (LBA4404 strain) containing the same cDNA in an anti-sense orientation. The plant of B- (3) is one emerged from the bud treated with water.
A.tumefaciens containing a 2.5 kb of buckwheat cDNA with unknown function in a sense orientation, while ones of another leaf from the same plant were inoculated by A.tumefaciens containing the same cDNA but in an anti-sense orientation. The inoculated leaves were incubated on wet vermiculite and then transferred to separate pots to grow to mature plants. As shown in Fig.1B, the plants emerged from the respective leaf exhibited phenotypes opposite to each other; the plants from the leaf inoculated by A.tumefaciens containing the cDNA in a sense orientation were tall and aged earlier, while the plants from the leaf inoculated by A.tumefaciens containing the cDNA in an anti-sense orientation were short and late in aging. From the result of the experiment, we were convinced that it is possible to transform plant by inoculating A.tumefaciens onto meristem of a plant, but not plant cell or tissue in vitro culture.
Seek for a model plant and transformation procedure
Kalanchoe plant seemed to have great advantages as a model plant for the in planta transformation; it has many big adventitious buds containing meristems on its leaf which are easy to access. Moreover, they are a clone. However, Kalanchoe plant has a fatal disadvantage; it is very difficult to obtain seeds. Thus, we sought for other plant as a model plant. Besides adventitious buds, meristem is included in various tissues or organs of any higher plant; it is included in a shoot, axillary bud, root tip and so on. We selected buckwheat (Fagopyrum esculentum var. Shinano No.1) as a model plant because it grows rapidly and produces many seeds within three months. Using buckwheat, we made up the in planta transformation procedure as follows (Kojima et al., 2000a; Kojima et al., 2000b). Buckwheat seeds sterilized with sodium hypochlorite were sown on soil in a pot and grown for 4 to 5 days to a stage of 7 to 8 cm height with two expanded leaves. Two to three points in top (0 to 3 mm part from top) of hypocotyl that included an apical meristem were pricked with a needle (φ 0.71
mm) and then inoculated with a cotton swab drenched with A.tumefaciens water suspension (ca 1.0 × 108 cells/ml). The inoculated seedlings were kept under the unfavorable conditions, at 220C in the dark, for 3 days to suppress defense action of plant so as to facilitate infection by A.tumefaciens. Subsequently, the seedlings were grown to maturation to set seeds (T1 generation) under non-sterilized and ambient conditions. Buckwheat plants develop two types of flowers with different pistil lengths, or hetrostylism. Only pollination between different types of flowers can result in fertilization. The flowers of plants in a pot were pollinated randomly by using a plumage.
Various plants such as mulberry (Morus alba L.), kenaf (Hibiscus cannabinus), rice (Oryza sativa), wheat (Triticum aestibvum L.), maize (Zea mays), and soybean (Glycine max) were successfully transformed by essentially the same method as one for buckwheat described above (Ping et al., 2003; Kojima et al., 2004; Suparthana et al., 2005; Suparthana et al., 2006; Kojima et al., 2006), except that A.tumefaciens was inoculated onto meristem of a different part of a plant among those plants. A. tumefaciens was inoculated onto meristems of axillary buds of young plants for mulberry, either apical or axillary buds of young plants for kenaf, apical buds of embryos in imbibed seeds for rice, wheat, and maize and axillary buds of decapitated seedlings for soybean.
Agrobacterium is eliminated by defense action of plant
In our transformation method, the inoculated immature plants did not receive any treatment to eliminate Agrobacteria. As for the inoculated imbibed seeds, they were treated once with antibiotics (Cefotaxime). It seemed unlikely, however, that Agrobacterium could be eliminated completely by such only one time antibiotic treatment. We, therefore, examined the presence of the remaining Agrobacteria used for transformation in the shoot apices of mature transformants in T0 and T1generations by the two experiments described below. No Agrobacteria
was detected in the shoot apices, which produce germ cells for the next generation.
In the conventional transformation methods, in which Agrobacteria are inoculated onto plant cells in vitro culture, it is very difficult to eliminate Agrobacteria completely. This may be because the conditions of in vitro culture are suitable not only for plant cells but also for Agrobacteria. On the other hand, under natural conditions, Agrobacterium is a rather week pathogenic bacterium; when Agrobacteria were inoculated onto leaves or stems of Kalanchoe plants grown in pots, galls emerged only on the inoculated sites, never on other parts of plants. From this result, the plants grown under such natural conditions appear to be resistant enough to confine Agrobacteria to the inoculated sites and eliminate them finally. Just like in the case above, the inoculated plants were also grown in pots under natural conditions in our in planta transformation method. Consequently, it is the most likely that Agrobacteria are eliminated by defense action of plant in our method.
In order to examine the presence of remaining Agrobacteria in the inoculated plants, we carried out two experiments. In the first experiment, shoot apices of mature plants in T0 and T1 generations were aseptically homogenized with autoclaved water using a mortar and pestle. The homogenates were inoculated onto LB media containing various antibiotics, on which Agrobacteria used for transformation could grow. The inoculated media were incubated at 28oC for 3 days. No colonies appeared for any homogenates, indicating the absence of Agrobacteria used for transformation. In the second experiment, genomic DNAs were isolated from shoot apices of mature plants in T0 and T1 generations and subjected to PCR analysis using them as templates. When the primer sets for genes in T-DNA region of binary vector were used, PCR products were obtained, while no PCR products were obtained when the primer sets for genes outside T-DNA region of binary vector were used. This result also indicated the absence of Agrobacteria used for transformation.
Plants of T0 generation are chimeras, while ones of T1 generation are true transformants
In our method, Agrobacteria were inoculated onto meristems of either apical or axillary buds of young plants or apical buds of embryos in imbibed seeds, depending on plants, in which various organs and primodia had already formed. Therefore, it is likely that the plants of T0 generation are chimeras. However, it is reasoned that transformants of T1 and subsequent generations produced by our method are not chimeric plants but truly transformed plants made from all transformed cells on the basis of the following consideration. Agrobacteria would likely transfer transgene not only to genomes of cells of meristems but also to genomes of already differentiated cells in buds. The transgenes integrated into genomes of these already differentiated cells could determine only phenotype of lower parts of mature T0 plants. In contrast, transgenes integrated into the genomes of as yet undifferentiated cells in meristems, which are destined to become apical meristems of mature plants, could contribute to production of shoot apices and hence to phenotype of mature T0plants. On maturation, germ cells (egg) and pollen (sperm) are produced by an apical meristem. An individual germ cell contains a single haploid copy of genomic DNA. Two germ cells, an egg and a sperm, produce a single fertilized cell, from which the entire body of cells of each transformant in the next generation is derived. Thus, transformants of T1 and subsequent generations should not be chimeric plants but true transformants. A considerable portion of T0 generation plants transformed by our method exhibited the phenotype different from that of nontransformed plants, being an indication that transgenes had been integrated efficiently in genomes of apical meristems of those mature T0 generation plants.
Based on the above consideration, we examined the transformation and integration of transgene in genomes of transformants using plants of T1 generation but not T0 generation in most of the experiments.
Demonstration of transformation
To allow transformation to be verified by various means, we used four different strains of A.tumefaciens, an avirulent M-21 mutant strain (Majumder et al., 2000), LBA4404 strain harboring a pBI 121 binary vector (Toyobo Co.), LBA4404 strain harboring a pIG121-Hm binary vector (Ohta et al., 1990) and LBA4404 strain harboring a binary vector (pBI-res2) modified for rescue of plasmid (Suparthana et al., 2006). M-21 strain is an avirulent mutant of which auxin synthesizing gene (iaaM) is destructed by Tn5-insertion, but all other genes including cytokinin synthesizing enzyme gene are intact. As a result, the transformants by M-21 strain were expected to synthesize a high level of cytokinin, resulting in a dramatically altered phenotype due to hormone imbalance, which is a good visible indication of transformation. Some altered phenotype also would be expected for transformants produced using other strains of A.tumefaciens since endogenous gene of plant might be destructed or modified by insertion of transgene into its genome. The second A.tumefaciens strain, LBA4404 strain with a pBI121 binary vector, is a standard strain for transformation of plants and contains a neomycin phosphotransferaseII (nptII) gene and a β-glucuronidase (GUS) gene. The third A.tumefaciens strain, LBA4404 strain with a pIG121-Hm binary vector, was used to indicate transformation by histochemical detection of β-glucuronidase as well as resistance to hygromycin B, since the pIB121-Hm binary vector contained a GUS gene with an intron and a hygromycin B resistance gene in its T-DNA. The fourth A.tumefaciens strain, LBA4404 strain with a pBI-res2 binary vector, was used to demonstrate definitively integration of transgene (T-DNA) in genome of transformant by rescuing the plasmid that contained integrated T-DNA and flanking plant chromosomal DNA in a contiguous segment. The plasmids were rescued from transformants of T1 generation as follows; genomic DNAs were extracted from transformants and digested with BamHI, which did not split the segment of
ampicilin resistance gene and replication origin of pBuescript plasmid in T-DNA region of the binary vector. The digested DNAs were selfligated and then transformed into E.coli. The transformed E.coli were screened on LB medium containing ampicilin and plasmids were isolated from E.coli grown on the medium. The structure of the isolated plasmid was determined by sequencing.
Many species of plants were transformed by using four strains of A.tumefaciens mentioned above and transformation was examined by various means. First, there appeared plants in T0 generation, which showed appearance different from that of parent plants, and their phenotype (appearance) was inherited by the progenies in the subsequent generations (Fig.2). Second, the seeds (T1 generation) of wheat transformants by LBA4404 strain harboring a pIG121-Hm binary vector showed hygromycin B resistance in their germination (Fig.3). Third, the wheat transformants (T1 generation) by A.tumefaciens LBA4404 strain harboring a pIG121-Hm binary vector showed positive β-glucuronidase activity (GUS) staining (Fig.4). Fourth, transgene integration into genome was demonstrated by Southern blot analysis with the wheat transformants (T1 generation) by A.tumefaciens LBA4404 strain harboring a pBI-res2 (Fig. 5). Fifth, PCR analysis detected transgene (GUS gene) in the genomes of buckwheat transformants (T1 generation) by A.tumefaciens LBA4404 strain harboring a pBI121 binary vector (Fig.6). Finally, the plasmids containing the integrated T-DNA and flanking genomic DNA in a contiguous segment were rescued from the T1 generation wheat transformants by A.tumefaciens LBA4404 strain harboring a pBI-res2 binary vector (Fig.7).
Transformation efficiency was calculated based on the results of the analyses described above. The transformation efficiency was from 40% to 60% with most of the plants tested such as buckwheat, rice and wheat. The transformation efficiency values of 40% to 60% seemed to be extremely high as compared with those by the conventional methods reported previously.
Fig. 2. Phenotypes of various species of transformants generated by the in planta transformation method. In the respective panel, the left side plants are nontransformants and the right side plants are transformants. (1) Buckwheat (Fagopyrum esculentum) transformants (T4 generation) by A.tumefaciens (LBA4404 strain) containing a rice MADS box gene (Acc. No. AB00325), (2) Mulberry (Morus alba L.) transformants (offspring produced from T0 transformants by stem cuttage) by A.tumefaciens (M-21 strain), (3) Kenaf (Hibiscus cannabinus) transformants (T1 generation) by A.tumefaciens (M-21 strain), (4) Rice (Oryza sativa) transformants (T1 generation) by A.tumefaciens (LBA4404 strain, pIG121-Hm), (5) Wheat (Triticum aestivum) transformants (T0 generation) by A.tumefaciens (M-21 strain), (6) Maize (Zea mays) transformants (T1 generation) by A.tumefaciens (LBA4404 strain, pIG121-Hm), (7) Soybean (Glycine max) transformants (T1 generation) by A.tumefaciens (M-21 strain)
However, such a high transformation efficiency obtained with our in planta method is reasonable in light of the experimental evidence and the reasons given below.
Reason for high transformation efficiency of our method
Kalanchoe plants (Kalanchoe daigremontiana) grown in a pot were inoculated on their stems or leaves with a virulent strain of A.tumefaciens
Fig. 3. Germination of seeds of the wheat transformants (T1 generation) by A.tumefaciens (LBA4404) strain harboring a pIG121-Hm binary vector in presence of hygromycin B (30 ppm). (A) germination of the transformants in presence of hygromycin B; (B) germination of the transformants in water; (C) germination of the nontransformants in presence of hygromycin B; (D) germination of the nontransformants in water (Suparthana et al., 2006).
Fig. 4. Histochemical assay of β-glucuronidase (GUS) in the wheat transformants (T1 generation) by A.tumefaciens LBA4404 strain harboring a pIG121-Hm
Fig. 5. Southern hybridization analysis of the wheat transformants (T1 generation) by A.tumefaciens LBA4404 strain harboring a pBI-res2 binary vector. Genomic DNAs of the transformants were digested with BamHI and SphI, which did not split the ori gene and ampicilin resistance gene of pBluscript plasmid segment in T-DNA region of the binary vector. The digested DNAs were fractionated on 0.8 % agarose gel and subsequently transferred to the membrane, which was probed with a Dig-labeled probe containing an ori gene. Lanes N1 and N2, different nontransformants; lane T1-T6, different transformants. The bottom band at 1.5 kb is not a band due to the integrated transgene but a band due to some segment of endogenous DNA of buckwheat genome (Suparthana et al., 2006).
Fig. 6. PCR detection of β-glucronidase gene in the buckwheat transformants (T1 generation) by A.tumefaciens LBA4404 strain harboring a pBI121 binary vector. The 1.2 kb DNA is a β-glucronidase gene segment amplified. M, size marker of DNA; lane 1, pBI121 binary vector; lane 2-4, different nontransformants; lane 5-24, different transformants (Kojima et al., 2000a).
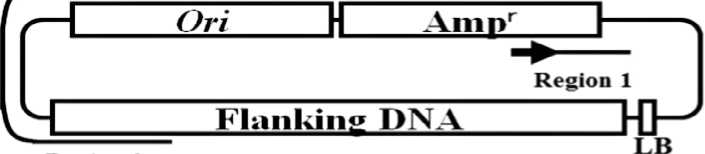
Region 2
Region 1
Upper sequence 5 sequence of region 1 Botrom sequence : sequence of corresponding region of bιnar>* vector
35 qgcocgctnc nqgcntccng cngicacgct cntπnocgg tatcqcnaca AAtuNrvncc ih i m ih i i ιιιιιιιι i i ii iiiiii ii i hi
≡er Ocaticctac aqccatcctc ctctcλccct Octcctttcc tatgoctka ttcacc-tcc
er Octtcccaac Gatcmoom agπacatca kccccatgt tgtgcaaaλa acocgπaoc ιιιιιιιιιι Iiiiiiiiii iiiiiiiiii Iiiiiiiiii iiiiiiiiii iiiiiiiiii zmo' Octicccaac gatcaagqcg agπacatga Tcccccatct igigcaaaaa agcggπaoc
κr κcττcocτc ckccatcct tckagaagt aagπgoccc cactcttak ackakcπ IIIIIIIIII IIIIIIIIII IIIIIIIIII IIIIIIIIII IIIIIIIIII IIIIIIIIII 8400’ kcttcogtc ckccatqgt tckagaagt aagπgqccg cagtgttatc ackakgh
zor Atgocaocac tqcataattc tctiactck atcocakcc tmgat-ttt ttπckact IIIIIIIIII IIIIIIIIII IIIIIIIIII IIIIIIIIII IIIIII Il Il lllllll
8400" atcqcaqcac tqcataattc tctiactck atcocakcc taacatcctt ttctckact
Region 2
Upper sequence J sequence of region 2
Bottom sequence ∑ sequence of wheat genomic DNA
(964C 10 2666retrotonspceen pro ten
TIGR «heat JenemeBLAST »earvw)
w; ci Hgacactcagiqggcgccc Iccgatgiaaqggacgacaacc Kaacgacatggiggc ozb Iiiiiiiiiiiiiiiiiiiiiiiiiiiiiiiiiiiiiiiiiiiiiiiiiiiiiiiiiiii
ZT CTΠCACACTCACTOCCCCCCCTCCCAKTAACCGACCACAACCKAACCACAKCTCGC W
wr Gcacggtattcttcccgcaaaagacakattgktqgcgtccagcatocgocgaagcatt zee ii ill iiiiiii inn iiiiiiiiii Iiiiiiiiiiiiiiiiii iiiiiiii GT CCαGQCCΠCΠCCAGCAAACGAGAKAΠCOGJOGCGKCAGCATOCGCTGAAGCAn 146
zor CccaacccctcacacacatCagckakgjΛτπτcτcATTKTκTatcocgqgtttre zee
ii inn iiiiiiii Iiiiiiiiiiiiiiiiii Iiiiiiiiiiiiiiii iiiii
Mr KCCAOCCCCCACACACACCΛGCKAKCJATΠTCΠAJTKTKTATCOCCGATΠK Z06
zor reπccaacctcaλqcikgkcqgcgλaπtkgλ:!κTATAa-KACTT-GCATAA iso Iiiiiiiiiii hi hi hi hi h ii ii iiiiii i i i ii hii i zor TC ΠCCAAOC TAAAGATK TKCACCiXATAT IACACTKTATCQCAnAGnKCAKA zee
MS KAGJAGT-GAAnc 1» I I I IIIIII
Z∙r CCIAAACTCCAAnC ZOl
Fig. 7. Assumed structure of a plasmid rescued from a wheat transformant (T1 generation) by A.tumefaciens LBA4404 strain harboring a pBI-res2 binary vector, and alignment of the determined sequence of the plasmid and the corresponding sequence of the assigned gene. The arrows indicate the positions and directions of the primers used for sequencing. The sequence of region 1 in the rescued plasmid was homologous with that of the corresponding region of the binary vector used for transformation, while the sequence of region 2 was homologous with that of a wheat genomic DNA (Acc. No. 9640 t02666, retrotransposon protein) (Suparthana et al., 2006).
(A-208 strain, a parent strain of M-21 avirulent strain used in this paper) by piercing with a needle previously smeared with A.tumefaciens and then the inoculated plants were continued to grow in a pot. After six weeks, galls were formed on every (100 %) inoculated site (Majumder et al., 2001). It has been revealed that gall formation results from integration of T-DNA region of Ti-plasmid indwelling in A.tumefaciens cell into plant genome, that is, “natural transformation”.
Some reasons are conceivable for that such an efficient natural transformation, gall formation, is accomplished under the conditions mentioned above. First, the factors necessary for gall formation such as auxin, cytokinin and other as yet unidentified factors might be provided at the best time, at the optimal concentrations and in the best combinations. Second, the meristems in the plants grown in a pot should have a stronger resistance to pathogen, A.tumefaciens
in this case, and stress and a higher capacity for differentiation and regeneration than cells or tissues in vitro culture. Finally, there might be significant roles in wounding of plant tissues that occurs on inoculation of A.tumefaciens. When wounded, plant should activate cell division to heal the wounded tissue. The activation of cell division must accompany activation of chromosome replication. Some special conditions allowing T-DNA to integrate effectively into plant chromosome might happen during the activated chromosome replication. Furthermore, the wounded plant also synthesizes many kinds of phenolic compounds and accumulates them in the wounded tissue and the surrounding tissue. The accumulated phenolic compounds have antimicrobial activity and so act as a chemical defense against pathogens. In addition, some of the phenolic compounds produced in response to wounding are reported to show the
same activity as acetosyringone; they induce the genes in vir region of Ti-plasmid or helper plasmid (Bolton et al., 1986). Consequently, the phenolic compounds in the wounded tissue might promote integration of T-DNA into plant genome.
There is close similarity between gall induction on Kalanchoe plants by A.tumefaciens mentionedabove,where“naturaltransformation” occurred on every (100 %) inoculated site, and our in planta transformation method; in the former case, the stems or leaves of Kalanchoe plants grown in pots were inoculated, accompanied by wounding, by A.tumefaciens and continued to grow in pots. In the later case, the meristems of immature plants were inoculated, accompanied by wounding, by A.tumefaciens and grown to maturation to set seeds in pots. The similarity between the two cases might account for the high transformation efficiency of our method.
Advantages of our in planta transformation method
Generally, the transformant generated by the in planta transformation method is superior to one by the conventional transformation method in that the former is the least likely accompanied with mutation since in vitro culture of plant sells, being prone to induce somatic mutation, is not involved in the process of the former.
In addition, our in planta method has four other advantages. First, it is simple as well as efficient. Third, we do not need to use a special A.tumefaciens strain; instead a standard and popular strain of A.tumefaciens, LBA4404 strain, is enough for our method. Finally, our method is applicable to wide species of plants. As shown in Fig.2, diverse species of crop plants have been successfully transformed by our method; they include dicotyledonous plants (buckwheat, kenaf and soybean), woody plant (mulberry) and monocotyledonous plants (rice, wheat and maize). It has been recognized that monocotyledonous crops, especially wheat and maize, are rather difficult to be transformed by the conventional transformation method using
A.tumefaciens (Hiei et al., 1994; Cheng et al., 1997). This possibly has to do with the phenomenon that crown gall disease is never seen with these monocotyledonous crops on the field. It is, therefore, noteworthy that monocot crops such as rice, wheat and maize were transformed with high efficiency by our in planta transformation method.
The remaining subject with plant transformation method
There remains an essentially important but unsolved problem with the method of plant transformation; that is the problem that we can neither designate location on the chromosome where the transgene should be integrated nor regulate the number of transgene to be integrated into chromosome. Such a present situation of plant transformation method is as if we were launching randomly a rocket without a control device toward plant cells. This situation is the case for both the conventional transformation methods and the in planta transformation methods. In order to solve this problem, we should develop a practical gene targeting method for plants as early as possible.
References
Bent AF. 2000. Arabidopsis in planta Transformation. Uses, Mechanisms, and Prospects for Transformation of Other Species. Plant Physiol. 124: 1540-1547
Bolton GW, Nester EW and Gordon MP. 1986. Plant phenolic compounds induce expression of the Agrobacterium tumefaciens loci needed for virulence. Science 232: 983-985
Chee PP, Fober KA and Slightom TL. 1989. Transformation of soybean (Glycine max) by infecting germinating seeds with Agrobacterium tumefaciens. Plant Physiol. 91:1212-1218
Cheng M, Fry JE, Pang S, Zhou H, Hironaka C, Duncan DR, Conner TW and Wan Y. 1997. Genetic transformation of wheat mediated by Agrobacterium tumefaciens. Plant Physiol.
115: 971-980
Clough SJ and Bent AF. 1998. Floral dip: a simplified method for Agrobacterium mediated transformation of Arabidopsis thaliana. Plant J. 16: 735-743
Hiei Y, Ohta S, Komari T and Kumashiro T. 1994. Efficient transformation of rice (Oryza sativa) mediated by Agrobacterium and sequence analysis of the boundaries of the T-DNA. Plant J. 6: 271-282
Hu CY and Wang L. 1999. In planta soybean transformation technologies developed in China: Procedure, confirmation and field performance. In Vitro Cell Dev. Biol. Plant 35: 417-420
Kojima M, Arai Y, Iwase N, Shiratori H, Shioiri H and Nozue M. 2000a. Development of a simple and efficient method for transformation of buckwheat plant (Fagopyrum esculentum)using Agrobacterium tumefaciens. Biosci. Biotechnol. Biochem. 64: 845-847
Kojima M, Hihara M, Koyama S, Shioiri H, Nozue M, Yamamoto K and Sasaki T. 2000b. Buckwheat transformed with cDNA of a rice MADS box gene is stimulated in branching. Plant Biotechnol. 17: 35-42
Kojima M, Shioiri H, Nogawa M, Nozue M, Matsumoto D, Wada A, Saiki Y and Kiguchi K. 2004. In planta transformation of kenaf plants (Hibiscus cannabinus var. aokawa no. 3) by Agrobacterium tumefaciens. J. Biosci. Bioeng. 98:136-139
Kojima M, Suparthana P, Teixeria da Silva JA and Nogawa M. 2006. Development of in planta transformation methods using Agrobacterium tumefaciens. In “Floriculture, Ornamental and Plant Biotechnology: Advances and Topical Issues VolumeII” Edited by Teixeira da Silva, J. A. Global Science Books. pp 42-48
Majumder P, Shioiri H, Nozue M and Kojima M. 2001. Isolation and characterization of new virulence gene (abv A) of Agrobacterium tumefaciens. J. Gen. Plant Pathol. 67: 124133
Majumder P, Yoshida H, Shioiri H, Nozue M and Kojima M. 2000. M-31 mutant (Vir A::Tn5) of Agrobacterium tumefaciens is capable of transferring its T-DNA into the nucleus of host cell, but incapable of integrating it into chromosome. J. Biosci. Bioeng. 90: 328-331
Ohta S, Mita S, Hattori T and Nakamura K. 1990. Construction and Expression in Tobacco of a 6- glucuronidase (GUS) Reporter Gene Containing an Intron Within the Coding Sequence. Plant Cell Physiol. 31: 805-813
Ping LX, Nogawa M, Nozue M, Makita M, Takeda M, Bao L and Kojima M. 2003. In planta transformation of mulberry trees (Morus alba L.) by Agrobacterium tumefaciens. J. Insect Biotechnol. Sericol. 72: 117-184
Qing CM, Fan L, Lei Y, Bouchenz D, Tourneur C, Yan L and Robaglia C. 2000. Transformation of Pakchoi (Brassica rapa L. ssp. chi-nensis) by Agrobacterium infiltration. Molecular Breeding 6: 67-72
Suparthana P, Shimizu T, Nogawa M, Shioiri H, Nakajima T, Haramoto N, Nozue M and Kojima M. 2006. Development of simple and efficient in planta transformation method for wheat (Triticum aestivum L.) using Agrobacterium tumefaciens J. Biosci. Bioeng. 102: 162-170
Suparthana P, Shimizu T, Shioiri H, Nogawa M, Nozue M and Kojima M. 2005. Development of simple and efficient in planta transformation method for rice (Oryza sativa L.) using Agrobacterium tumefaciens. J. Biosci. Bioeng. 100: 391-397
Touraev A, Stoger E, Voronin V and Heberle-Bors E. 1997. Plant male germ line transformation. Plant J. 12: 949-956
udayana university biosciences and biotechnology forum • 59
Discussion and feedback