IN SILICO STUDY OF Hibiscus Sabdariffa Linn. ACTIVE COMPOUNDS IN GLP-1R: POTENTIAL AS ANTIDIABETIC DRUG
on
JURNAL KIMIA (JOURNAL OF CHEMISTRY) 17 (2), JULI 2023 DOI: https://doi.org/10.24843/JCHEM.2023.v17.i02.p2
p-ISSN 1907-9850
e-ISSN 2599-2740
IN SILICO STUDY OF Hibiscus Sabdariffa Linn. ACTIVE COMPOUNDS IN GLP-1R: POTENTIAL AS ANTIDIABETIC DRUG
T. Andriani1, N. Mawaddah2*, L. Erlina3, R. K. Anggraeni2, N. Ibrahim1, M. Siagian1
-
1Department of Medical Physiology and Biophysics, Faculty of Medicine, Universitas Indonesia, Jakarta 10430, Indonesia
-
2Master’s Program in Biomedical Sciences, Faculty of Medicine, Universitas Indonesia, Jakarta 10430, Indonesia 3Department of Chemistry, Faculty of Medicine, Universitas Indonesia, Jakarta 10430, Indonesia
*Email: [email protected]
ABSTRAK
Diabetes melitus merupakan gangguan metabolisme yang ditandai dengan kondisi hiperglikemia akibat produksi insulin yang berkurang dan atau kondisi resistensi insulin. GLP-1 dapat meningkatkan sekresi, sensitivitas insulin, dan menurunkan kadar glukosa darah dengan cara mengikat reseptor GLP-1 (GLP-1R). Beberapa tanaman telah digunakan sebagai obat antidiabetes. Penelitian ini bertujuan untuk melihat bagaimana interaksi senyawa aktif dalam Hibiscus sabdariffa Linn. terhadap GLP-1R secara in silico. Penelitian dimulai dengan melakukan validasi internal reseptor GLP-1 (6ORV) kemudian dilakukan proses docking terhadap native ligand. Hasil validasi reseptor 6ORV mempunyai nilai RMSD 2.14Å. Hasil score docking 3 senyawa aktif Hibiscus sabdariffa Linn. terbaik adalah myricetin-3-arabinogalactoside (-10,64 kcal/mol), tetra-O-methyljeediflavanone (-9,95 kcal/mol), dan ethyl chlorogenate acid (-7,73 kcal/mol). Asam amino yang berperan terhadap afinitas pada sisi aktif ikatan ligan dengan reseptor adalah Trp 297, Trp 203, Met 204, Phe 230, Try 220, Lys 197. Temuan ini menunjukkan bahwa senyawa aktif Hibiscus sabdariffa Linn. dapat berikatan langsung dengan GLP-1R.
Kata kunci: Diabetes melitus, Hibiscus sabdariffa Linn., GLP-1R
ABSTRACT
Diabetes mellitus is a metabolic disorder characterized by hyperglycemia due to reduced insulin production and/or insulin resistance. GLP-1 can increase insulin secretion, improve insulin sensitivity, and lower the blood glucose levels by binding to the GLP-1 receptor (GLP-1R). Several plants have been used as antidiabetic drugs. This study aims to see the interactions of the active compounds in Hibiscus sabdariffa Linn. and GLP-1R in silico. The research started with internal validation of the receptor GLP-1 (6ORV), and then the native ligand was docked. The 6ORV receptor validation results have an RMSD value of 2.14Å. The results of docking scores of the 3 best active compounds Hibiscus sabdariffa Linn. are myricetin-3-arabinogalactoside (-10.64 kcal/mol), tetra-O-methyljeediflavanone (-9.95 kcal/mol), and ethyl chlorogenate acid (-7.73 kcal/mol). The amino acids that contribute to the affinity at the active site of the ligand bond with the receptor are Trp 297, Trp 203, Met 204, Phe 230, Try 220, Lys 197. These findings indicate that the active compound Hibiscus sabdariffa Linn. can bind directly to GLP-1R.
Keywords: Diabetes mellitus, Hibiscus sabdariffa Linn., GLP-1R
INTRODUCTION
Diabetes mellitus (DM) is a metabolic disorder characterized by hyperglycemia due to reduced insulin production, insulin resistance, or both.(Biessels & Reagan, 2015) In 2019, the International Diabetes Federation (IDF) estimated that there were 463 million
individuals with DM, and this number is projected to increase to 578 million in 2030 and 700 million in 2045.(Daniels & Mietlicki-Baase, 2019) Indonesia is included in the IDF Asia-Pacific region, with 19.5 million DM cases found in Indonesia in 2021.(International Diabetes Federation, 2021)
DM causes various complications, often associated with long-term damage, dysfunction, and failure of various organs.(Kodl & Seaquist, 2008) The most common complications included persistent kidney failure (nephropathy), nerve injury (neuropathy), which increases the likelihood of ulcers and is the main cause of amputation of the lower extremities, eye disorders (retinopathy) which causes blindness, coronary heart failure, diseases involving peripheral arteries, cognitive impairment, and cerebrovascular disorders.(Saeedi et al., 2019) Some efforts are needed to treat patients appropriately in order to reduce the morbidity and mortality due to its complications.
Management of DM requires multidisciplinary action, which includes pharmacological and non-pharmacological therapy. The high incidence and prevalence of DM encourage researchers to improve and develop better therapy for DM patients that can reduce the risk of complications and increase the quality of life. Incretin-based therapy for DM is currently being developed.(Soelistijo, 2021) The effect of incretin is a response to release more insulin due to high postprandial blood glucose levels, which is responsible for 50-70% of total insulin secretion after oral glucose administration. The incretin effect is played by two hormones, namely GLP-1 and GIP.(Baggio & Drucker, 2007)
Glucagon Like Peptide-1 (GLP-1) is one of the incretin hormones produced from the preproglucagon (PPG) gene and secreted by enteroendocrine L cells in response to the presence of glucose in the intestine.(Müller et al., 2019) GLP-1 shows various positive effects, such as increasing insulin secretion and sensitivity and lowering blood glucose levels.(Cho et al., 2014) Other beneficial effects of DM conditions include delayed gastric emptying, maintenance of pancreatic β cells (proliferation, neogenesis, antiapoptosis), inhibition of food intake and energy consumption, and function in increasing glucose uptake by muscle cells.(Fujiwara et al., 2019) Interestingly, GLP-1 also has antiinflammation and antioxidant activity, and also has a positive impact on other organs such as cardiovascular and brain, and organs affected by DM complications. GLP-1 mediates its effects on various organs by binding to the GLP-1 receptor (GLP-1R) which is expressed in its related organs. GLP-1 and GLP-1R bonds
will activate this receptor and produce certain cellular signaling and corresponding physiological responses.(Yildirim Simsir et al., 2018)
Glucagon Like Peptide-1 receptor agonist is a new class of therapy for DM. This type of drug is represented by Exenatide, Liraglutide, Exenatide LAR, Albiglutide, and Tasphoglutide. Despite its superiority that can naturally mimic human GLP-1, of course there are side effects that patients can experience due to its consumption, including causing nausea, vomiting, constipation, diarrhea, bloating, and other digestive disorders.(Trujillo et al., 2021)(Wharton et al., 2022) Besides that, these drugs are still rare and sold at relatively high prices.(Reid, 2012)
Currently, many studies have discussed the potential of new active compounds from natural ingredients, including those derived from plants to be developed as new drug. Indonesia is known as a country with high biodiversity, marked by the many plants that can be used in medicine, one of which is Hibiscus sabdariffa Linn. or Rosella which is known to have the potential to prevent DM and its complications.(Guardiola & Mach, 2014) Hibiscus sabdariffa Linn. is rich in bioactive compounds such as anthocyanins, quercetin, other flavonoids, organic acids, and polysaccharides, which have several beneficial physiological effects, such as antioxidant, antibacterial, anti-inflammatory, anticholesterol and anti-diabetic effects.(Jabeur et al., 2017)
The active compounds of herbal medicines are believed to have lower side effects, high bioavailability, and low cytotoxic effects.(Umadevi et al., 2022) Candidates for a new drug in diabetes mellitus in the botanical sector must start with an in silico study. In silico studies have been widely used to search for and design new potential drugs from natural materials and are also expected to optimize the research process and prevent losses in vitro and in vivo research steps in a precise, accurate, fast, and effective manner. The mechanism of antidiabetic effect of Hibiscus sabdariffa Linn. could be through GLP-1 receptor activation. Therefore, this study aims to see how the interactions of the active compounds in Hibiscus sabdariffa Linn. against GLP-1R in silico are.
MATERIALS AND METHOD
Materials and Tools
The materials used consisted of the three-dimensional structure of GLP-1R obtained through the Protein Data Bank website (PDB ID = 6ORV) and 39 compounds derived from the Hibiscus sabdariffa Linn. plant. This research used MarvinSketch, AutoDockTools, Ligplot+, and PyMOL software. The Software was operated on Lenovo ThinkPad with i7-4600U processor, 8GB RAM, Windows 13, and 64-bit operating system.
Ligands Preparation.
Ligands are the phytochemical constituents of Hibiscus sabdariffa Linn. from a study by (Herranz-López et al., 2017) as many as 39 compounds were added with N2V as the control positive ligand. Two and threedimensional ligand structures were obtained from the Pubchem® database in .sdf format. Structures that were not found in the Pubchem® database were drawn manually using MarvinSketch. All structures were optimized in three dimensions and changed the format to .pdb using MarvinSketch. All these ligands were then given charge, set torsion and written as pdbqt format using AutoDockTools-1.5.6.
Protein Preparation
The three-dimensional structure of the GLP-1R was downloaded from the Protein Data Bank (PDB ID: 6ORV). Furthermore, the unused part including water molecules was removed, hydrogen nonpolar was added, gasteiger charged was given, and Grid box size and grid center coordinate were arranged. A grid box of 40 x 50 x 40 Å was generated around the center of the active site residues of receptor. This structure was then converted to pdbqt format. All these steps were conducted using AutoDockTools-1.5.6.
Validation of Molecular Docking Protocol
The main objective of molecular docking is to identify energetically favorable binding modes of ligands into the target receptor’s binding site.(Ece, 2018) Validity is determined by redocking the native ligand to a prepared target protein. The method is said to be valid if the Root Mean Square Deviation (RMSD) value obtained is ≤ 2Å.(Puratchikody et al., 2016) To ensure that the tested ligand binds in the ideal position for each ligand, the
binding site orientation is carried out using the blind docking method, and the results of all tested ligands show cavity with the highest affinity equal to the comparative ligands. Docking search parameter is used by the Lamarckian genetic algorithm with the number of genetic algorithms being 10 runs, population size is 150, the maximum number of energy evaluating is medium which is 2.500.000, the maximum number of generations is 27.000, spacing default is 0.375 Å, and the default docking parameter is used for running options.
The primary parameters used in the docking process are the free energy of binding (∆G), the inhibition constant (Ki), amino acid residues, and the number of hydrogen bonds.(Kim et al., 2016) ∆G and Ki scores determine ligand affinity to the receptor. The more negative the ∆G and the lower the Ki scores indicate higher ligand affinity toward the active site of the receptor. All tested ligands are then compared with the result of control positive ligand to determine the potency of both tested ligands.(Natesan et al., 2012) The amino acid residues of ligands are then compared with amino acid residues of control positive ligand to assess the similarity of interaction between ligands and control positive ligand. More similar amino acid residues indicate a higher probability that the ligand will have a similar activity to the control positive ligand.(Pena Neshich et al., 2015) Visualization of twodimensional and three-dimensional ligandreceptor interactions was performed using Ligplot+ and PyMOL software.
RESULTS AND DISCUSSION
Molecular docking is a computational research method that aims to estimate the interaction and affinity of a ligand with a macromolecule (usually an enzyme or protein). A ligand and protein molecule are predicted by placement techniques in a specific area (an active site) to provide optimal results (Motiejunas & Wade, 2006). The molecular docking method uses the AutoDock 4.2.6 software application. Docking validation is used successively for the x center (125.194), y center (109.599), and z center (81.738) with an RMSD value obtained from the method validation results of 2.14Å. Molecular docking results show an interaction between the active compounds Hibiscus sabdariffa Linn. which
interact with GLP-1R and have an activating interacting with GLP-1R are described in the
function (as an activator). The active table below.
compounds Hibiscus sabdariffa Linn.
Table 1. The Results Of The Comparison Between ΔG And Ki Values From The Docking Of The Active Compounds Hibiscus sabdariffa Linn. With The GLP-1R
No |
Compound Name |
Binding Energy (kcal/mol) |
Inhibition Constant |
1 |
1-O-caffeoylquinic acid |
-5.80 |
55.63 uM |
2 |
2-O-caffeoylhydroxycitric acid |
-4.19 |
847.27 uM |
3 |
2-O-trans-Feruloylhydroxitric acid |
-7.08 |
6.41 uM |
4 |
3-Methylquercetin |
-5.83 |
52.93 uM |
5 |
Coumaroylquinic acid |
-5.65 |
72.28 uM |
6 |
5-O-Caffeoylshikimic acid |
-5.58 |
81.63 uM |
7 |
Caffeoylglucose |
-3.18 |
4.65 mM |
8 |
Chlorogenic acid |
-4.88 |
263.31 uM |
9 |
Chlorogenoquinone |
-5.99 |
40.85 uM |
10 |
Cryptochlorogenic acid |
-4.60 |
424.20 uM |
11 |
Cyanidine 3-sambubioside |
-5.42 |
106.68 uM |
12 |
Delphinidin 3-O-beta-D-sambubioside |
-5.57 |
83.26 uM |
13 |
Delphinidin 3-sambubioside |
-5.43 |
104.28 uM |
14 |
Ethyl chlorogenate acid |
-7.73 |
2.16 uM |
15 |
Hibiscus acid |
-2.40 |
17.48 mM |
16 |
Hibiscus acid dimethylester |
-3.60 |
2.29 mM |
17 |
Hydroxycitric acid |
-2.61 |
12.19 mM |
18 |
Kaempferol |
-5.74 |
61.85 uM |
19 |
Kaempferol-3-Glucuronide |
-5.91 |
46.21 uM |
20 |
Kaempferol-3-O-sambubioside |
-5.91 |
46.65 uM |
21 |
Kaempferol-3-O-rutinoside |
-6.98 |
7.62 uM |
22 |
Leucoside |
-5.55 |
86.03 uM |
23 |
Methyl digallate-para |
-7.13 |
5.98 uM |
24 |
Methyl diga llate-meta |
-7.30 |
4.48 uM |
25 |
Methyl-epigallocatechin |
-7.65 |
2.47 uM |
26 |
N-Feruloyltyramine |
-6.47 |
18.04 uM |
27 |
Myricetin |
-5.25 |
142.32 uM |
28 |
Myricetin-3-arabinogalactoside |
-10.64 |
15.82 nM |
29 |
Myricetin-3-glucoside |
-5.34 |
122.59 uM |
30 |
Neochlorogenic acid |
-5.38 |
112.93 uM |
31 |
Prodelphinidin-B3 |
-5.81 |
55.54 uM |
32 |
Quercetin |
-5.62 |
75.38 uM |
33 |
Quercetin 3-7-diglucuronide |
-5.75 |
60.49 uM |
34 |
Quercetin 3-O-glucuronide |
-6.13 |
31.95 uM |
35 |
Quercetin 3-rutinoside |
-7.12 |
6.09 uM |
36 |
Quercetin 3-sambubioside |
-5.11 |
178.74 uM |
37 |
Quercetin-3-Glucoside |
-5.53 |
89.01 uM |
38 |
Tetra-O-methyljeediflavanone |
-9.95 |
50.48 nM |
39 |
Hibiscus acid hydroxyethylesther |
-4.41 |
582.73 uM |
40 |
Native Ligand |
-12.02 |
1.53 M |
Bond-free energy is a vital aspect for assessing the interaction between the ligand and the receptor. This energy indicates the bond strength (affinity) between the tested ligand and the target protein. The more negative the value of the bond energy obtained, the more stable the complex formed is between GLP-1R (6ORV) and the active compound derived from Hibiscus
sabdariffa Linn. Increasingly negative values indicate that the compound has an interaction in the form of increasing attraction between atoms. In contrast, the repulsive forces between particles become minimal so that the conformation of the compound obtained is more stable (Manna et al., 2017). The low bond energy value also indicates that less energy is
required to carry out the binding or interaction of a ligand with a receptor. Meanwhile, the inhibition constant is directly proportional to the number of doses needed to cause pharmacological activity. The smaller the inhibition constant, the smaller the required dose is (Vargas et al., 2018). Several factors affect the binding affinity between the ligand and the receptor. Electrostatic, van der Waals, and hydrophobic interactions are important factors besides other factors such as entropy, desolvation, flexibility of the receptor structure, and the structure of the water molecule at the bond site (Haider, 2010).
In this study, three active compounds were found and had the best docking results, namely ethyl chlorogenate acid with ∆G (-7.73 kcal/mol) and Ki (2.16 uM), myricetin-3-arabinogalactoside with ∆G (-10.64 kcal/mol) and Ki (15.82 nM), and tetra-O-methyljeediflavanone with ∆G (-9.95 kcal/mol) and Ki (50.48 nM). These three compounds have the closest free bond energy to the positive control ligand value and have the highest binding affinity among the other ligands. The analysis results also obtained amino acids which are thought to play a role in the affinity of the active compounds of Hibiscus sabdariffa Linn. to GLP-1R, as seen in Table 2.
The target binding sites of this protein are located at Trp 297, Trp 203, Met 204, Ala 200, Leu 201, Leu 217, Phe 230, Try145, Try148, Try220, Lys 197. The essential amino acid compounds contained in the positive control ligand, namely Myricetin-3-arabinogalactoside, Tetra-O-methyljeediflavanone, and Ethyl chlorogenate acid, which are the three active compounds with the best docking, and they are Trp 297, Trp 203, Met 204, Phe 230, Try 220, Lys 197. Even though it differs from the position of the positive control ligand hydrogen bond (N2V), the presence of hydrogen bonds in the active compound with the best docking value has a vital role in the affinity between the compound and the target protein because it forms electrostatic interactions (donor and acceptor hydrogen) (Muttaqin, 2019). The amino acid residue plays a role in activating GLP-1R to carry out its function. The determined distance between the bonds indicates that the active compound has bonded with amino acid residues. In general, bonds occur at a distance of about 5 Ǻ and not below 1 Ǻ because there will be repulsions between atoms so that it does not meet the general bonding requirements (Faridah et al., 2021).
Table 2. Comparison Between Amino Acid Residues And The Number Of Hydrogen Bonds | ||||
Amino Acid |
Native Ligand |
Myricetin-3-arabinogalactoside |
Tetra-O-methyljeediflavanone |
Ethyl chlorogenate acid |
Trp 297 |
4 |
4 |
4 |
4 |
Trp 203 |
4 |
√ |
√ |
4 |
Met 204 |
4 |
4 |
4 |
4 |
Ala 200 |
√ |
√ |
- |
4 |
Leu 201 |
4 |
4 |
- |
- |
Leu 217 |
√ |
- |
√ |
4 |
Phe 230 |
4 |
4 |
4 |
4 |
Try 145 |
√ |
(H) 3.29 Å |
- |
- |
Try 148 |
√ |
- |
- |
- |
Try 220 |
(H) 3.09 Å |
√ |
√ |
4 |
Lys 197 |
(H) 2.65 Å |
√ |
(H) 3.26 Å |
4 |
Cys 226 |
- |
- |
√ |
4 |
Cys 296 |
- |
- |
√ |
4 |
Val 229 |
- |
√ |
√ |
4 |
Met 233 |
- |
√ |
- |
4 |
Asp 198 |
- |
4 |
- |
- |
Val 194 |
- |
- |
- |
- |
The visualization of interactions between the top three ligands and GLP-1R used Ligplot and PyMOL. The comparison between the molecular interactions of the top three
ligands and the interactions of the positive control ligand molecules towards the same receptor is shown in Figures 1 and 2.
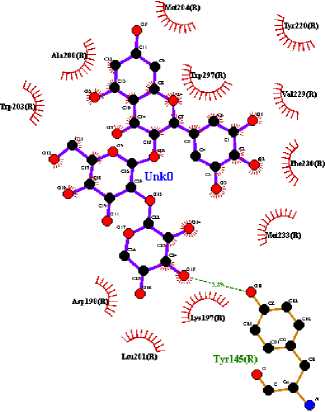
(b)
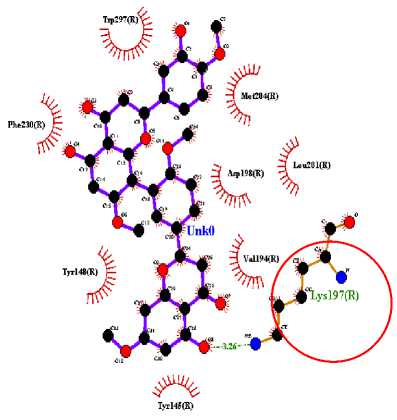
(c)
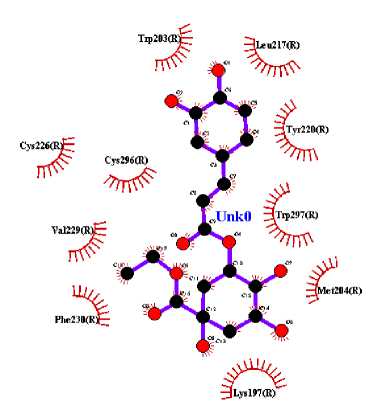
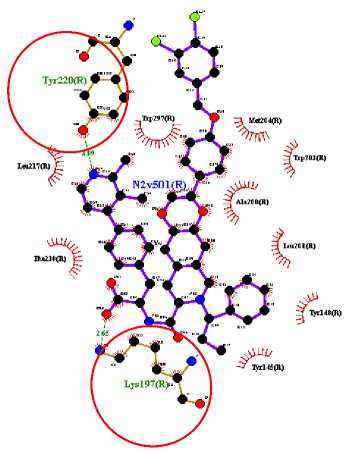
Figure 1. Results Of 2D Molecular Docking Visualization Of The Top Three Active Compounds And GLP-1R (a) Myricetin-3 arabinogalactoside And GLP-1R; (b) Tetra-O-methyljeediflavanone And GLP-1R; (c) Ethylchlorogenate acid And GLP-1R; (d) Native Ligand And GLP-1R
(a)
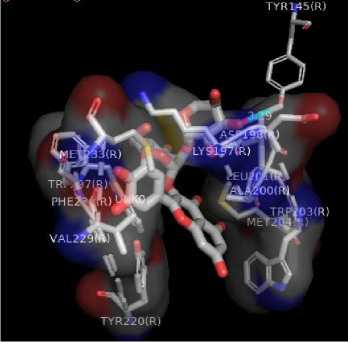
(b)
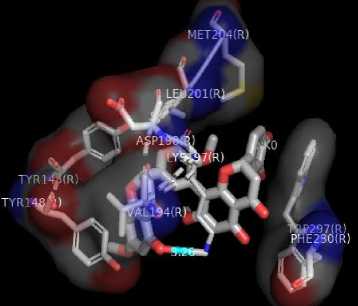
(c)
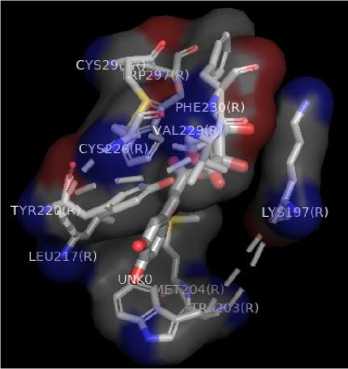
Figure 2. Results Of 3D Molecular Docking Visualization Of The Top Three Active Compounds And GLP-1R (a) Myricetin-3 arabinogalactoside And GLP-1R; (b) Tetra-O-methyljeediflavanone And GLP-1R; (c) Ethylchlorogenate acid And GLP-1R; (d) Native Ligand And GLP-1R
(d)
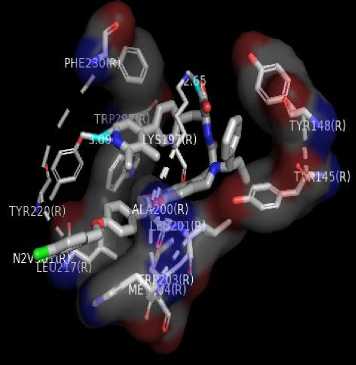
The compounds with the top three docking results were then examined for their physicochemical properties to see the potential of the compounds when developed into medicinal preparations. The physicochemical parameters of the tested compounds were adjusted according to the Lipinski rule. There are five rules: (1) if the test compound has a molecular weight of more than 500 Da, it will be difficult for the compound to penetrate the cell membrane; (2) a log P value greater than 5 indicates that the compound is increasingly lipophilic and binds very tightly to the membrane, making it challenging to identify targets and is also toxic; (3) However, if the log
P value is too small or negative, it is also not very good because it will be difficult for the compound to penetrate the lipid bilayer membrane; (4) Hydrogen bond donors and acceptors are the magnitudes of the hydrogen bond capacity; (5) If the hydrogen capacity is higher, the energy required in the absorption process is also higher. In general, the Lipinski rule of five (RO5) indicates the solubility of certain compounds in penetrating the membrane by passive diffusion (Brito, 2011). The test compounds are said to meet the requirements for the formation of oral preparations if there is no more than one violation of the Lipinski rules (Lipinski, 2004).
Table 3. Results Of The Lipinski Rule Of Five
Ligan |
Molecular Weight (g/mol) |
HydrogenB ond Acceptor |
Hydrogen Bond Donor |
LogP |
Description |
Myricetin-3-arabinogalactoside |
612.49 |
17 |
11 |
-1.91 |
Did not meet Lipinski RO5 requirements |
Tetra-O-methyljeediflavanone |
614.60 |
11 |
3 |
4.31 |
Did not meet Lipinski RO5 requirements |
Ethyl chlorogenate acid |
382.36 |
9 |
5 |
0.21 |
Met Lipinski RO5 requirements |
Based on Table 3, of all the top active compounds analyzed, there was one compound that met the Lipinski RO5 requirements and met the requirements for making oral preparations. Further research is needed to determine the pharmacokinetic profile of these active
compounds. Then the active compound must have a good ADME profile and be non-toxic, and the top three test compounds will be evaluated for their toxicity through the AdmetSAR website (Cheng et al., 2012).
Table 4. Toxicity Test Results
Ligand |
Toxicities | ||
AMES |
Carcinogenesis |
AOT | |
Myricetin-3-arabinogalactoside |
- |
- |
III |
Tetra-O-methyljeediflavanone |
- |
- |
III |
Ethyl chlorogenate acid |
- |
- |
III |
Table 5. Ligand Pharmacokinetic Observations | |
Ligand |
Pharmacokinetics Absorption Inhibitor CYP GI CYP1A2 CYP2CI9 CYP2C9 CYP2D6 CYP3A4 |
Myricetin-3-arabinogalactoside |
Low No No No No No |
Tetra-O- methyljeediflavanone |
Low No No Yes No No |
Ethyl chlorogenate acid |
Low No No No No No |
According to the results of the analysis, the top three active compounds are not carcinogenic, non-toxic, and included in the AOT III category, which means they are slightly toxic if ingested orally. Then the three observed ligands show that these ligands have low gastrointestinal absorption. Gastrointestinal absorption can be an indicator of whether oral administration of the compound will be effective or not. Interactions of the compounds with cytochrome P450 (CYP1A2, CYP2C19, CYP2C9, CYP2D6, CYP3A4) were identified. These iso-enzymes play a major role in drug elimination through metabolic biotransformation. If this enzyme is inhibited,
the drug or its metabolites may accumulate in the body, causing toxic effects (Yang et al., 2017). Myricetin-3-arabinogalactoside and Ethyl chlorogenate acid are predicted to be noninhibitors for one of the isoenzymes. However, Tetra-O-methyljeediflavanone turned out to be a CYP2C9 inhibitor.
This study shows the potential of the active compound Hibiscus sabdariffa Linn. to interact with GLP-1R. This interaction might cause activation of the GLP-1R and initiate signal transduction and produce associated physiological responses. For example, pancreatic β cells activates several signals that have an impact on insulin secretion, increasing
proliferation, and preventing apoptosis of pancreatic β cells (Ma et al., 2014)(Kartinah et al., 2019). Hibiscus sabdariffa Linn. has ability to maintain circulating GLP-1 levels to reach its target in the pancreas (Domínguez Avila et al., 2017). This in silico study showed an additional beneficial effect of Hibiscus sabdariffa Linn. whose active compounds can also act as a ligand independent variable which is predicted to have antidiabetic activity by binding to GLP-1R.
CONCLUSION
Based on the docking results of 39 test compounds on Hibiscus sabdariffa Linn. three candidate compounds with the best binding affinity with GLP-1R were obtained, namely myricetin-3-arabinogalactoside, tetra-O-
methyljeediflavanone, and ethyl chlorogenic acid. Based on visualization of the interaction of active compounds in GLP-1R, it can be concluded that amino acids play a role in the active site of the receptor, namely Trp 297, Trp 203, Met 204, Phe 230, Try 220, and Lys 197. However, the results of this study must be validated further through both in vitro and in vivo studies to elucidate the potential activity through GLP-1 and GLP-1R which produce the expected responses in diabetes mellitus management.
REFERENCE
Baggio, L. L., & Drucker, D. J. 2007. Biology of Incretins: GLP-1 and GIP.
Gastroenterology. 132(6): 2131–2157.
https://doi.org/10.1053/j.gastro.2007.03.0 54
Biessels, G. J., & Reagan, L. P. 2015.
Hippocampal insulin resistance and cognitive dysfunction. Nature Reviews Neuroscience. 16(11): 660–671.
https://doi.org/10.1038/nrn4019
Brito, M. A. de. 2011. Pharmacokinetic study with computational tools in the medicinal chemistry course. Brazilian Journal of Pharmaceutical Sciences. 47(4): 797–
805. https://doi.org/10.1590/S1984-
82502011000400017
Cheng, F., Li, W., Zhou, Y., Shen, J., Wu, Z., Liu, G., Lee, P. W., & Tang, Y. 2012. admetSAR: A Comprehensive Source and Free Tool for Assessment of Chemical ADMET Properties.
https://doi.org/10.1021/ci300367a
Cho, Y. M., Fujita, Y., & Kieffer, T. J. 2014. Glucagon-Like Peptide-1: Glucose
Homeostasis and Beyond. Annual Review of Physiology. 76(1): 535–559.
https://doi.org/10.1146/annurev-physiol-021113-170315
Daniels, D., & Mietlicki-Baase, E. G. 2019. Glucagon-like peptide 1 in the brain: Where is it coming from, where is it going? Diabetes. 68(1): 15–17.
https://doi.org/10.2337/dbi18-0045
Domínguez Avila, J. A., Rodrigo García, J., González Aguilar, G. A., & de la Rosa, L. A. 2017. The Antidiabetic Mechanisms of Polyphenols Related to Increased Glucagon-Like Peptide-1 (GLP1) and Insulin Signaling. Molecules. 22(6): 1–16. https://doi.org/10.3390/molecules220609 03
Ece, A. 2018. E-Pharmacophore Mapping Combined with Virtual Screening and Molecular Docking to Identify Potent and Selective Inhibitors of P90 Ribosomal S6 Kinase (RSK). Turkish Journal of
Pharmaceutical Sciences. 13(2): 241– 248. https://doi.org/10.4274/tjps.28290
Faridah, F., Sumaryono, W., Simanjuntak, P., & Triwibowo, R. R. 2021. Analysis of Pancreatic Lipase Inhibitor Activity of Chlorogenic Acid Derivatives in Green Coffee Beans as Antiobesity using In Silico. Jurnal Ilmu Kefarmasian Indonesia. 19(1): 125.
https://doi.org/10.35814/jifi.v19i1.946
Fujiwara, Y., Eguchi, S., Murayama, H., Takahashi, Y., Toda, M., Imai, K., & Tsuda, K. 2019. Relationship between diet/exercise and pharmacotherapy to enhance the GLP‐1 levels in type 2 diabetes. Endocrinology, Diabetes & Metabolism. 2(3): 1–14.
https://doi.org/10.1002/edm2.68
Guardiola, S., & Mach, N. 2014. Therapeutic potential of Hibiscus sabdariffa: A review of the scientific evidence. Endocrinología y Nutrición (English Edition). 61(5), 274– 295.
https://doi.org/10.1016/j.endoen.2014.04. 003
Haider, M. K. 2010. Computational Analysis of Protein-Ligand Interaction. In Yuki Gosei Kagaku Kyokaishi/Journal of Synthetic Organic Chemistry. University of York. https://doi.org/10.5059/yukigoseikyokais hi.54.427
Herranz-López, M., Olivares-Vicente, M., Encinar, J., Barrajón-Catalán, E., Segura-Carretero, A., Joven, J., & Micol, V. 2017. Multi-Targeted Molecular Effects of Hibiscus sabdariffa Polyphenols: An Opportunity for a Global Approach to Obesity. Nutrients. 9(8): 907.
https://doi.org/10.3390/nu9080907
International Diabetes Federation. 2021. International Diabetes Federation. In Diabetes Research and Clinical Practice (10th ed., Vol. 102, Issue 2). https://doi.org/10.1016/j.diabres.2013.10. 013
Jabeur, I., Pereira, E., Barros, L., Calhelha, R. C., Soković, M., Oliveira, M. B. P. P., & Ferreira, I. C. F. R. 2017. Hibiscus sabdariffa L. as a source of nutrients, bioactive compounds and colouring agents. Food Research International. 100: 717–723.
https://doi.org/10.1016/j.foodres.2017.07. 073
Kartinah, N. T., Fadilah, F., Ibrahim, E. I., & Suryati, Y. 2019. The Potential of Hibiscus sabdariffa Linn in Inducing Glucagon-Like Peptide-1 via SGLT-1 and GLPR in DM Rats. BioMed Research International. 2019: 1–8.
https://doi.org/10.1155/2019/8724824
Kim, J., Kwon, J., Kim, M., Do, J., Lee, D., & Han, H. 2016. Computational protein– ligand docking and virtual drug screening with the AutoDock suite. Polymer
Journal. 48(7): 829–834.
https://doi.org/10.1038/pj.2016.37
Kodl, C. T., & Seaquist, E. R. 2008. Cognitive Dysfunction and Diabetes Mellitus.
Endocrine Reviews. 29(4): 494–511.
https://doi.org/10.1210/er.2007-0034
Lipinski, C. A. 2004. Lead- and drug-like compounds: The rule-of-five revolution. Drug Discovery Today: Technologies. 1(4): 337–341.
https://doi.org/10.1016/j.ddtec.2004.11.0 07
Ma, X., Guan, Y., & Hua, X. 2014. Glucagon-like peptide 1-potentiated insulin secretion and proliferation of pancreatic β-cells. Journal of Diabetes. 6(5): 394–402.
https://doi.org/10.1111/1753-0407.12161
Manna, A., Laksitorini, M. D., Hudiyanti, D., & Siahaan, P. 2017. Molecular Docking of Interaction between E-Cadherin Protein and Conformational Structure of Cyclic
Peptide ADTC3 (Ac-CADTPC-NH2) Simulated on 20 ns. Jurnal Kimia Sains Dan Aplikasi,. 20(1): 30–36.
https://doi.org/10.14710/jksa.20.1.30-36
Motiejunas, D., & Wade, R. C. 2006. Structural, energetic, and dynamic aspects of ligandreceptor interactions. Comprehensive Medicinal Chemistry II. 4: 193–212.
https://doi.org/10.1016/b0-08-045044-x/00250-9
Müller, T. D., Finan, B., Bloom, S. R., Alessio, D. D., Drucker, D. J., Flatt, P. R., & Fritsche, A. 2019. Glucagon-like peptide 1 (GLP-1). Molecular Metabolism. 30(September): 72–130.
https://doi.org/10.1016/j.molmet.2019.09. 010
Muttaqin, F. Z. 2019. Molecular Docking and Molecular Dynamic Studies of Stilbene Derivative Compounds As Sirtuin-3
(Sirt3) Histone Deacetylase Inhibitor on Melanoma Skin Cancer and Their
Toxicities Prediction. Journal of
Pharmacopolium. 2(2): 112–121.
https://doi.org/10.36465/jop.v2i2.489
Natesan, S., Subramaniam, R., Bergeron, C., & Balaz, S. 2012. Binding affinity prediction for ligands and receptors forming tautomers and ionization species: Inhibition of mitogen-activated protein kinase-activated protein kinase 2 (MK2). Journal of Medicinal Chemistry. 55(5): 2035–2047.
https://doi.org/10.1021/jm201217q
Pena Neshich, I., Nishimura, L., de Moraes, F., Salim, J., Villalta-Romero, F., Borro, L., Yano, I., Mazoni, I., Tasic, L., Jardine, J., & Neshich, G. 2015. Computational Biology Tools for Identifying Specific Ligand Binding Residues for Novel Agrochemical and Drug Design. Current Protein & Peptide Science. 16(8): 701– 717.
https://doi.org/10.2174/13892037166661 50505234923
Puratchikody, A., Sriram, D., Umamaheswari, A., & Irfan, N. 2016. 3 ‐ D structural interactions and quantitative structural toxicity studies of tyrosine derivatives intended for safe potent inflammation treatment. Chemistry Central Journal. 1– 19. https://doi.org/10.1186/s13065-016-
0169-9
Reid, T. 2012. Choosing GLP-1 Receptor Agonists or DPP-4 Inhibitors: Weighing
the Clinical Trial Evidence. 30(1): 3–12. https://diabetesjournals.org/clinical/articl e/30/1/3/35430/Choosing-GLP-1-Receptor-Agonists-or-DPP-4
Saeedi, P., Petersohn, I., Salpea, P., Malanda, B., Karuranga, S., Unwin, N., Colagiuri, S., Guariguata, L., Motala, A. A., Ogurtsova, K., Shaw, J. E., Bright, D., & Williams, R. 2019. Global and regional diabetes prevalence estimates for 2019 and projections for 2030 and 2045: Results from the International Diabetes Federation Diabetes Atlas, 9th edition. Diabetes Research and Clinical Practice. 157: 107843.
https://doi.org/10.1016/j.diabres.2019.10 7843
Soelistijo, S. 2021. Pedoman Pengelolaan dan Pencegahan Diabetes Melitus Tipe 2 Dewasa di Indonesia 2021. Global Initiative for Asthma, 46.
Trujillo, J. M., Nuffer, W., & Smith, B. A. 2021. GLP-1 receptor agonists: an updated review of head-to-head clinical studies. 1– 15.
https://doi.org/10.1177/20420188219973 20
Umadevi, P., Manivannan, S., Fayad, A. M., & Shelvy, S. 2022. In silico analysis of phytochemicals as potential inhibitors of proteases involved in SARS-CoV-2 infection. Journal of Biomolecular Structure and Dynamics. 40(11): 5053– 5059.
https://doi.org/10.1080/07391102.2020.1 866669
Vargas, J. A. R., Lopez, A. G., Piñol, M. C., & Froeyen, M. 2018. Molecular docking study on the interaction between 2-substituted-4,5-difuryl Imidazoles with different protein target for antileishmanial activity. Journal of Applied
Pharmaceutical Science. 8(3): 14–22.
https://doi.org/10.7324/JAPS.2018.8303
Wharton, S., Davies, M., Dicker, D., Lingvay, I., Mosenzon, O., Rubino, D. M., &
Pedersen, S. D. 2022. Managing the gastrointestinal side effects of GLP-1 receptor agonists in obesity: recommendations for clinical practice. Postgraduate Medicine. 134(1): 14–19.
https://doi.org/10.1080/00325481.2021.2 002616
Yang, H., Chaofeng, L., Lixia, S., Jie, L., Yingchun, C., Zhuang, W., Weihua, L., Guixia, L., & Yun, T. 2017. AdmetSAR 2.0: web-service for prediction and
optimization of chemical ADMET properties. Bioinformatics. 33(16),: 1–7. https://doi.org/10.1093/bioinformatics/bt y707/5085368
Yildirim Simsir, I., Soyaltin, U. E., &
Cetinkalp, S. 2018. Glucagon like peptide-1 (GLP-1) likes Alzheimer’s disease.
Diabetes and Metabolic Syndrome:
Clinical Research and Reviews. 12(3): 469–475.
https://doi.org/10.1016/j.dsx.2018.03.002
128
Discussion and feedback