DNA MICROARRAY BASED EXPRESSIONAL PROFILING OF HRPXO DEPENDENT UP-REGULONS IN XANTHOMONAS ORYZAE PV. ORYZAE
on
ISSN: 9 772303 337008
ARTICLE
DNA MICROARRAY BASED EXPRESSIONAL PROFILING OF HRPXO DEPENDENTUP-REGULONS IN XANTHOMONAS ORYZAE PV. ORYZAE
Byoung Ho So1, Jongun Kim1, Hee Wan Kang1,2*
1Graduate School of Biotechnology and Information Technology, Hankyong National University, Ansung 456-749, Korea
2Institute of Genetic Engineering, Hankyong National University, Ansung 456-749, Korea
*Corresponding author: Hee-Wan Kang
Graduate School of Biotechnology and Information Technology, Hankyong National University, Ansung 456-749, Korea
Tel: +82-31-670-5420; Fax: +82-31-676-2602; E-mail: kanghw2@hknu.ac.kr
ABSTRACT
Thirty-mer oligonucleotides for microarray analysis were designed from the annotated open reading frames (ORFs) in the whole genome sequence of X. oryzae pv. oryzae KACC10331. Mutant hrpXo::TN and wild-type strain KACC10859 were cultured in hrp inducing medium (XOM2), and cDNAs, which were synthesized from the total RNA samples from both strains, were hybridized on a DNA microarray. The microarray data showed that 210 genes were down-regulated more than 2-fold in hrpXo::TN, while 115 genes were up-regulated more than 2-fold. The HrpXo regulons differently included 54 hypothetical genes: type III secretory genes (11 hrp genes); genes encoding type III secretory effectors (xopP genes and avr/pthA); genes encoding type II secretory effectors (6 proteases, a lipase, a polygalacturonase, and 4 cellulases); 7 iron-uptake genes; 6 pil genes encoding fimbrial assembly membrane proteins; and 14 transposon genes. Significant plant-inducible promoter (PIP) sequences were newly identified on HrpXo regulons. The validity of the expressional profiles was further confirmed by reverse transcription (RT)-PCR.
INTRODUCTION
Xanthomonas oryzae pv. oryzae (Xoo), a causal agent of bacterial blight (BB), is economically the most important bacterial pathogen in rice plants (Oryza sativa L.) worldwide. The hypersensitive response and pathogenicity (mediated by the hrp gene) and the production of extracellular polysaccharides (EPS), extracellular enzymes, and lipopolysaccharides (LPS) are known to be critical factors in pathogenesis (Chan and Goodwin 1999; Shen and Ronald 2002). Additionally, various virulence genes have been newly identified from the Xoo genome by transposon mutagenesis (Park et al. 2007; Wang et al. 2008).
The hrp genes encode proteins involved in the type III secretion system (T3SS), which is involved in the secretion of effector proteins from bacteria to plant (Büttner and Bonas 2002; Grulebeck et al. 2005; Keyu et al. 2005). The hrp gene cluster in Xoo is composed of 27 genes, from hrpa2 to hrpF (Lee et al. 2005), and the expression of these genes is regulated by 2 regulators, hrpG and hrpX, which are separate from the hrp gene
cluster (Oku et al. 1995; Tsuge et al. 2005). Hrpg belongs to the OmpR family and activates the expression of hrpX, an AraC-like transcription activator that controls hrp genes along with some effector proteins (Tsuge et al. 2006; Wengelnik and Bonus 1996; Wengelnik et al. 1999). The HrpX regulons of Xanthomonas species have been found to include a consensus sequence motif termed the plant-inducible promoter region (PIP) box (TTCGC-N15-TTCGC) around the promoter regions (Furutani et al. 2006; Koebnik et al. 2006; Tsuge et al.2005). In Xoo, an additional conserved cis-regulatory element, a -10 boxlike sequence that resembles the binding motif of RNA polymerase, was found downstream of the PIP box (Furutani et al. 2006). By using PIP box sequences, numerous candidate genes belonging to Hrp regulons, other than the genes in the hrp cluster, have been identified from the Xanthomonas genome database (da Silva et al. 2002; Furutani et al. 2006; Koebnik et al. 2006). It has been demonstrated that HrpXo regulates the transcriptional expression of genes associated with type II secretory proteins such as cysteine proteases (Furutani et al. 2004). Recently, it was
reported that polygalacturonase (PG) Wanga) et al.2008; and extracellular proteases (Wei et al. 2007) in X. campestris pv. campestris are regulated by HrpX. These reports suggest that HrpX can potentially function in regulating the expression of other genes in addition to the hrp genes. A useful hrp-inducing medium, XOM2, was established for cultivation of Xoo and has greatly contributed to elucidating the in vitro functional roles of hrpXo (Tsuge et al. 2002).
Xanthomonas genomes, including the genomes of Xoo, X. axonopodis pv. citri, and X. campestris pv. campestris, have been completely sequenced (da Silva et al. 2002; Lee et al. 2005; Ochiai et al. 2005), and our knowledge of the sequences provides a powerful insight into the general and specific features of pathogenicity-related genes in Xanthomonas genomes. On the basis of the sequence information, DNA microarrays of the Xanthomonas oryzae pathovars oryzae and oryzicola have been constructed as biosensors, which can be used for high-throughput genome-wide gene expression analysis to study the regulation of hrp genes (Seo et al. 2008). In addition, DNA microarrays of the phytopathogenic bacteria Pseudomonas syringae, Ralstonia solanacearum, Xanthomonas axonopodis, Xanthomonas campestris, and Xylella fastidiosa have been used to probe genome-wide expression related to pathogenesis and to assess the genomic diversity of meaningful bacterial isolates by (Astua-Monge et al. 2005; Guidot et al. 2009; He et al. 2007; He et al. 2006; Koide et al. 2006; Lu et al. 2005; Wang et al. 2006). DNA microarray chip technology promises to allow monitoring of the entire genome on a single chip, thereby providing researchers with a better picture of the simultaneous interactions among thousands of genes (de Morgan et al. 2010).
In this study, our aim was to identify the genome-wide hrpXo regulons by using an Xoo DNA microarray. The Xoo microarray was constructed using 30-mers derived from annotated open reading frames (ORFs) in the genome sequence of Xoo KACC10331. The transcriptional profiles of the hrpXo mutant
were compared with those of the wild-type strain KACC10859. The resultant hrpXo regulons included different genes, including T3SS genes, T3SS effector genes, type II secretory system (T2SS) effector genes such as genes encoding proteases and cellulases, as well as genes involved in the uptake of iron, fimbrial-assembly genes, and metabolism-related genes.
MATERIALS AND METHODS
Bacterial strains and culture
Wild type strain, X. oryzae pv. oryzae KACC10859 was obtained from the Korean Agricultural Culture Collection (KACC) at the National Academy of Agricultural Science (NAAS), Suwon, Korea. Transposon mutant, hrpXo::TN were a gift from Dr. Cho of National Academy of Agricultural Science, Suwon. Xoo strains were cultured on pepton sucrose agar (PSA) medium (Tsuchiya et al. 1982) at 28°C for 3 days. Antibiotic, kanamycin were added to final concentration (20 mg/liter) 20 for mutant, hrpXo:: TN.
Induction of hrpXo gene expression
Method of Tsuge et al. (2002) was applied to modification for inducing hrpXo gene expression. Wild type and mutant, hrpXo::TN strains were grown on PSA for 3 days and the bacterial cells were washed twice distilled water (DW) and resuspended in DW at an optical density of 2.0 /ml at 600 nm. The bacterial cells were harvested by centrifugation at 6,000 rpm and suspended in 5 ml of XOM2 medium and incubated with shaking for 18 h at 25 C. The cells were used for total RNA extraction.
Fabrication of DNA microarray
Fifty-mer oligonucleotides were designed from the respective ORFs in the genomic sequence data of X. oryzae pv. oryzae KACC10331 by employing the following criteria: melting temperatures were normalized within 2°C; the G+C content of the designed oligonucleotides was restricted to 50 ± 5%, matching the 63.7%
G+C content of X. oryzae pv. oryzae KACC10331; “no sequence homology” to other regions of the genome was restricted to a maximum of 35 bp, with no exact sequence matches of more than 15 bp. Finally, 3,057 oligonucleotides were directly synthesized on CombiMatirx CustomArray™ 12K Microarray (Ghindilis et al. 2007)according to the manufacturer’s protocol (Macrogen, Korea).
cDNA labeling and hybridization
The experiments were performed according to the standard CombiMatrix protocol described in detail at). Total RNA from mutant hrpXo::TN and the wild-type strain KACC10859, which were grown on XOM2 media, was purified by using the RNeasy mini kit in accordance with the manufacturer’s instructions (Qiagen, Valencia, CA, U.S.A.). Labeled cDNA was synthesized by random primed reverse transcription reactions in the presence of Cy3/Alexa 546 or Cy5/Alexa 647 by using SuperScript II (Invitrogen, USA). One microgram of RNA was mixed with 2 μg of random primers, heated to 80°C for 10 min, and cooled on ice for 2 min. Unlabeled deoxynucleoside triphosphates (10 mM each of dATP, dTTP, dGTP, and dCTP), 200 units of reverse transcriptase, 5× reverse transcription buffer, and 1 μl of SUPERase-In RNase inhibitor (Ambion, U.S.A.) were added to a final volume of 20 μl. The samples were incubated at 42°C for 2 h. The reaction was stopped by the addition of 3.5 μl of stop-solution (0.5 M NaOH, 50 mM EDTA), and the RNA was degraded by incubation at 65°C for 15 min. The mixture was neutralized by adding 5 μl of 1 M Tris-HCl (pH 7.5), and 21.5 μl of 1× TE buffer was added to a final volume of 50 μl. After purification of the cDNA sample by using a MinElute PCR purification kit (Qiagen, Germany), 16 μl of the sample was heated to 80°C for 10 min and cooled on ice for 2 min. Four microliters of 10 mM dTTP, either 2.5 μl of terminal deoxynucleotidyl transferase, and 2.5 μl of 10× tailing buffer were added to a final volume of 25 μl. The samples were incubated at 37°C for 30 min, heated to 95°C for 10 min, and then immediately cooled on ice for 2 min.
Five microliters of 6× ligation mix (Cy3/Alexa 546 or Cy5/Alexa 647) and 2 μl of T4 DNA ligase (Genishpere, U.S.A.) were added to a final volume of 32 μl. The sample was incubated at room temperature for 30 min, and 14.5 μl of 1× TE buffer was added to a final volume of 50 μl.
For hybridization, 2× hybridization buffer (6× SSPE, 0.05% Tween-20, 20 mM EDTA, 5× Denhardt’s solution, 1 mg Salmon sperm DNA, and 0.05% SDS) was added to the CombiMatirx CustomArray™ 12K Microarray assembly and prehybridized at 65°C for 10 min, and 10 μl of the labeled cDNA was added to a prewarmed microarray and then incubated at 65°C overnight in a dark humidified chamber. After hybridization, the microarrays were washed with pre-warmed washing buffer A (6× SSPE), B (3× SSPE), and C (0.5× SSPE) at 42°C for 15 min each and finally washed with PBS buffer.
Image scan
ElectraSenseTM Reader (http://www. combimatrix.com/ products electrasense.htm) and ElectraSenseTM 12K microarrays were used for all electrochemical assays according to the standard CombiMatrix protocol. The microarrays were assembled with special ElectraSenseTM caps to create hybridization chambers. After hybridization, the microarrays were washed and incubated with HRP-Avidin solution in BSA Peroxidase Stabilizer (1:1000) for 45 min. Finally, the hybridization chambers were filled with TMB solution, and the microarrays were inserted into the ElectraSenseTM Reader for detection.
Reverse transcriptase-PCR analysis
The mutant strain hrpXo::TN and wild-type strain KACC10859 were cultured in 5 ml of the hrp-inducing medium XOM2 (Tsuge et al., 2002) and were extracted with an RNeasy kit (Qiagen, Valencia, CA, U.S.A.), which was followed by DNase I (Promega, U.S.A.) treatment. The RNA concentration was determined using a NanoDrop photometer (NanoDrop, Wilmington, DE, U.S.A.). Primer sets designed from the 67 genes were used for reverse transcriptase
(RT)-PCR analysis to verify the hrpX-regulated genes appearing on the DNA microarray. After the quality of the RNA was confirmed on a 1% agarose gel, it was added to a 20-μl reaction mixture containing 20 pmol of downstream primer, 4 μl of 5× RT buffer, 2 μl of dNTPs (10 mM), 10 units of RNase inhibitor, and 10 units of reverse transcriptase, and incubated in a PTC-225TM thermocycler (MJ Research, USA) under the following conditions: 42°C for 30 min. After the cDNA synthesis reaction, 10 μl of each cDNA was PCR-amplified by HQ Taq Polymerase (JK Biotech. Korea) using the gene-specific primer sets in Table 1 under the following conditions: 35 cycles of 94°C for 30 sec, 60°C for 30 sec, and 72°C for 1.5 min. The PCR amplicons were visualized by ethidium bromide staining after 1% agarose gel electrophoresis. As a positive control, 16S rRNA was used. The primer set was as follows: 16S_F, 5′-GGAGGAACATCAGTGGCGAAGG-3′ and 16S_R, 5′-CCGAAGGCACCAATCCATCTCTG-3′.
RESULTS AND DISCUSSION
Microarray data analysis
In this study, we aimed to perform genomewide identification of hrpXo regulons in Xoo by using DNA microarray analysis. The XOM2 media was formulated to induce the expression of hrp genes, including hrpX, and has been used to study hrpX-dependent expression in the Xoo genome (Furutani et al. 2004; Tsuge et al. 2002). Therefore, we employed this medium to induce expression of the hrpX gene. Labeled cDNAs were synthesized from the total RNA from hrpXo::TN and the wild type strain KACC10859, which were cultured in XOM2, and the synthesized cDNAs were hybridized with the Xoo DNA microarray plate with 3 duplications. Oligonucleotides consisting of 30 bases were designed from the annotated ORFs in the whole genome sequence data of Xoo KACC10331, but ORFs with length less than 50 bp and those with repetitive sequences, such as transposable elements, were excluded from this study. Finally,
individual oligonucleotides designed from the 3,017 ORFs were synthesized on the microarray. The expression profile of the wild-type strain KACC10859 differed from that of the hrpXo:: TN mutant strain (Fig. 1 A), and the differences were observed in the 3 duplicated samples. Fig. 1B shows a scatter plot of the up- and down-regulated genes in hrpXo::TN, when compared with the expression in the wild-type strain. A large number of genes were down-regulated. In total, 210 genes were down-regulated more than 2-fold in the hrpXo::TN mutant, while 115 genes were up-regulated, suggesting that numerous hrpXo-regulated genes are distributed in the Xoo genome. The oligonucleotides are directly synthesized on individually addressable microelectrodes in a semiconductor matrix, and therefore, a relatively fixed quantity can be provided for microarray analysis.
In a previous study, the hrp-inducing XVM2 medium, which provides an environment similar to the host intercellular components, was extensively used for inducing virulence genes in different Xanthomonas species (Wengelnik et al. 1996). A DNA microarray consisting of 279 potential pathogenicity-related virulence genes from X. axonopodis pv. citri was applied for comparison between the transcriptomes from X. axonopodis pv. citri colonies cultured in XVM2 and nutrient broth media (Gustavo et al. 2005). When X. axonopodis pv. citri was grown in XVM2 medium, 31 genes, including those encoding cell wall degradation enzymes, Hrps, and avirulence proteins were profiled to be up-regulated, indicating that the artificial media can induce the expression of pathogenicity-related genes. The XOM2 medium was modified for Xoo with the same objective and mainly used for studying hrpX-regulated genes (Tsuge et al. 2002). In addition, microarray analysis to compare the global gene expression in representative strains of Xoo and X. oryzae pv. oryzicola (Xoc) grown in PSB and XOM2 has been performed (Seo et al. 2008). It was found that 247 Xoo genes and 39 Xoc genes, including bacterial-motility and signal-transduction related genes, hrp, and rax
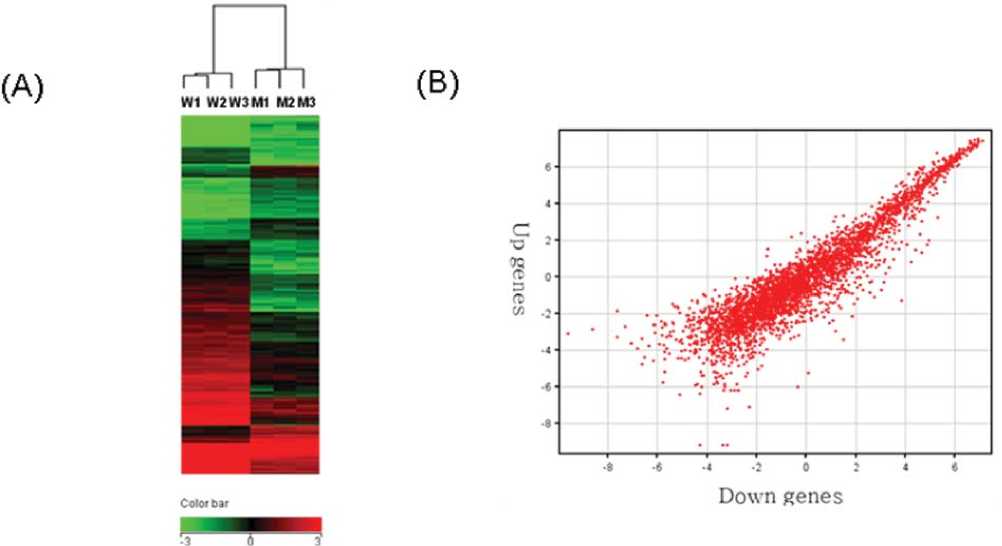
Fig 1. Expression profiling (A) of the hrpX::TN mutant and X. oryzae pv. oryzae KACC10859 on DNA microarray, and scatter plot (B) of hrpX regulatory genes in the hrpX::TN mutant compared to wild-type strain. M: mutant strains; W: wild-type strains. The scale indicates the relative level of expression of each gene, where red indicates expression and green indicates repression.
genes, are up-regulated in XOM2. Nevertheless, it was reasonably considered that the artificial XOM2 medium can provide environmental signals for inducing different genes, such as metabolism-related genes as well as hrp genes, under different media conditions. However, we aimed to determine how hrpX induces target genes by comparing the expressional profiles of the hrpX mutant and wild-type strains under the same induction medium, XOM2. Thus, we believe that the down-regulated genes on the mutant microarray are dependent on hrpX expression, although their expression intensities are different.
To date, different hrpX-induced genes have been identified by the presence of a PIP box, a cis-regulatory element with a motif [TTCGC-N15-TTCGC] that exists in promoter regions of HrpX regulons. The PIP box has been used as effective marker for screening HrpX regulons (Furutani et al. 2006; Koebnik et al. 2006). Genome-wide screening for PIP boxes was performed on the whole genomic sequence of Xoo (Furutani et al.
2006; Lee et al. 2005). On the basis of the PIP box sequences, 17 HrpXo regulons were identified in the genome sequence of Xoo KACC10331. Of these, the cysteine proteinase gene, iron receptor gene, and hrp genes were coincident to those observed in the present study. Furthermore, in this study, we investigated the existence of PIP box sequences in the hrpXo-regulated genes on a microarray. As shown in Fig. 2, perfect PIP boxes, containing the motif TTCGT- N15-TTCGT, were identified in the promoter regions of 4 hrp genes (XOO0095, XOO0096, XOO0094, and XOO0076). Genes encoding cysteine protease contained imperfect PIP boxes (TTCGn-N12-TTCGn in XOO0387, TTCGC-N12-TTCGC in XOO1480, and TTCGT-N13-TTCGT in XOO1487) in their promoter regions. Significant PIP boxes were further identified in other hrpXo-regulated genes, including the xrvA gene of virulence regulator (XOO2744), a cellulase gene (XOO4036), and 2 pili genes (XOO1157, XOO1158), but not in the other hrpX regulons in this study. In Xanthomonas species, genes with an imperfect PIP box and
XOOO387 XOO1480 |
TTCGC TTCGC |
tgcctcagcgct------ tgcctcagcgct------ |
TTCGC TTCGC |
Cystein protease |
XOO1487 |
TTCGT |
aagcggcatcgcc----- |
TTCGT | |
XOO2744 |
TTCGC |
gcagcgtgtccactctta |
TTCCG |
Xtv A; viru Ience regulator |
XOO4036 |
TTGTC |
tccacccgcacaggccac |
TTCGC |
cellulase |
X001157 |
TTGCC |
tagcgcgtgaagggc--- |
TTGTC |
pilM |
XOO1158 |
TTCGC |
ggtcgaaaacgcc----- |
TTCGC |
P∣IN |
X000095 |
TTCGC |
gcgtacaagcgcaat--- |
TTCGC |
hrp1 |
X000096 |
TTCGC |
ttgcccgttaagtgt--- |
TTCGT |
hrp2 |
XOO0094 |
TTCGC |
Gagcgacttccgata--- |
TTCGC |
hrcC |
XOO0076 |
TTCGC |
cacaccagctatcgc--- |
TTCGC | |
PIP |
TTCGC |
-N15- |
TTCGC |
hrpE |
Fig. 2. PIP sequences in the promoter regions of hrpXo-regulated genes on the X. oryzae pv. oryzae microarray. The window boxes indicate consensus sequences in PIP (standard sequence).
genes without a PIP box have been reported to be expressed in an HrpX-dependent manner (Noël et al. 2002), suggesting that the PIP box is not an absolute criterion for identifying HrpXo regulons.
hrpXo dependent up-regulated genes Pathogenesis-related genes
Virulence and regulatory genes required for bacterial pathogenicity are commonly found in pathogenicity islands (PAIs) that encode for a type III protein secretion system assembled from hrp gene products. During infection, phytopathogenic bacteria use T3SS to deliver effector proteins into the host plant, thereby causing disease symptoms in susceptible hosts and defense responses in resistant hosts. An hrp gene cluster containing 27 genes, including hpa2 and hrpF, was identified in the Xoo genome. The Xoo hrp PAI (31.3 kb) was larger than its counterparts in Xac (25.6 kb) and Xcc (23.1 kb), owing to the presence of 4 transposed genes (about 6 kb) located between the hpaB and hrpF genes (Lee, et al. 2005). In this result, it was revealed that hrpXo regulates the transcriptional expression of hrpF, hrpE, hrpD6, hpaA, hrcV, hrpB3, hrpB8, hrcC, hpa1, and hpa2 (Table 1). Previously,
microarray experiments were performed to compare global gene expression between Xoo and Xoc grown in nutrient medium (PSB) and those grown in hrp-inducing XOM2 media (Seo et al., 2008). The hpa1 and hrpE genes in X. oryzae pv. oryzicola are specifically induced in hrp-inducing XOM2 media, but not in nutrient media, indicating that hpa1 and hrpE are under different or additional regulatory controls from the other hrp genes, which is unlikely to be the case in Xoo. In contrast, expression of Xoo hpa1, hrpG, hrcC, and hrpE were up-regulated in XOM2. However, it is not clear whether the induced expression of the hrp genes in XOM2 is due to environmental signals in the media or hrpXo. On the basis of our data, we considered that hrpX expression is induced by environmental factors in plants or XOM2 media and that the activated HrpX then stimulates the expression of other hrp genes such as hpa1, hrpG, hrcC, and hrpE. HrpX is known to be a regulator of hrp genes and several effector genes (Wengelnik and Bonas 1996).
Furthermore, we identified 3 T3SS effector genes XOO4466 (XopP), XOO2127 (Avr/PthA), and XOO4033 (XopP) in this microarray analysis. Xoo harbors multiple genes for T3S effectors of the transcription activator-like (TAL) effector family,
called the avrBs3/pthA family (Lee et al. 2005; Ochiai et al. 2005). AvrXa7, AvrXa10, and AvrXa27 are representative TAL effectors that elicit disease resistance in hosts with corresponding resistance genes and thereby function as determinants of race–cultivar specificity (Gu et al. 2005; Yang et al. 2000). Hrp outer proteins (Hops) from P. syringae strains and Xanthomonas outer proteins (Xops) have been shown to be T3S effectors. Recently, on the basis of the natural features of N-terminal amino acids of Hop, Xop proteins, and T3S substrates, T3S system-dependent translocation of 60 effector candidates was identified from the genome data of Xoo MAFF311018 and screened using Bordetella pertussis calmodulin-dependent adenylate cyclase (Cya) reporter for translocation into plant cells (Frutani et al. 2008). Finally, 16 T3SS effector genes that are also regulated by hrpX were identified. Of these, XOO4466 and XOO4033, from this study, were included among the effectors.
The rtx genes (XOO4535 and XOO1389) have been shown to be up-regulated by HrpXo in Bradyrhizobium elkanii, a symbiotic nitrogenfixing bacterium that produces the enol-ether amino acid rhizobitoxine; the rtxC gene is required for the production of rhizobitoxine, which is regarded as a phytotoxin responsible for progressing chlorosis symptoms in soybean (Okazaki et al. 2004). RTX toxins are important virulence factors for a variety of human and animal pathogens (Welch et al. 1992) and have been found in several plant pathogenic bacteria, including X. fastidiosa, Rhizobium leguminosarum, and Erwinia carotovora (Kuhnert et al. 1997; Oresnik et al. 1999; Simpson et al. 2000). The genes for 2 apparent RTX toxins, rtxA and rtxC, were identified in the Xoo genome but were not detected in the Xcc or Xac genomes.
The virulence gene xrvA, which encodes a histone-like nucleoid-structuring protein, was also revealed to be an hrpXo regulon. Recently, it was reported that xrvA demonstrates enhanced expression in XOM2 medium and further regulates pathogenicity-related genes such as hrpG, hrpX, rpfC, and rpfG, which play an
important role in the hypersensitive response and EPS and LPS biosynthesis (Feng et al. 2009). The xrvA function found in the study by Feng et al. seems to be contrary to the result of our microarray analysis, but this is not conclusive because we do not know how virulence genes are interracially associated with other unknown pathogenesis-related genes.
In the present study, it was revealed that 7 iron-receptor proteins, which encode the genes XOO4431, XOO0407, XOO0008, XOO0009, XOO0029, and XOO1368, are regulated by hrpXo. In X. campestris pv. campestris, TonB, ExbB, and ExbD have been reported to play a critical role in Fe3+ uptake and induction of a hypersensitive response in pepper (Wiggerich et al. 1997; Wiggerich and Puhler 2000). Forty-two putative iron uptake-related genes, including TonB-dependent receptor proteins, were annotated in the genomic sequence of Xoo KACC10331. In a recent report, mutation in a TonB-dependent receptor led to the loss of pathogenicity and also caused a reduction in mobility and extracellular enzymes (Wu et al. 2010). A mutation in XOO1368, homologous to bfeA, resulted in reduced pathogenicity (Wang et al. 2008), suggesting that sequestration of iron is important for Xoo virulence. A 3-gene cluster (bfeABR) is also present in the Xoo genome. The bfeA gene was isolated from Bordetella pertussis and Bordetella bronchiseptica, obligate respiratory pathogens of mammals that encode a predicted outermembrane siderophore receptor exhibiting a high degree of similarity to the ferric enterobactin receptors. The extremely limited availability of free iron requires pathogenic bacteria to utilize specialized uptake mechanisms to successfully compete with the host and other microorganisms for iron.
Table 1. Downregulated genes in hrpX: TN mutant strain
Gene ID |
Description |
Expression folds |
Gene ID |
Description |
Expression folds |
XOO0832 |
holC;DNA polymerase III subunit chi |
-2.02 |
XOO1623 |
rpsT;30S ribosomal protein S20 |
-2.58 |
XOO4019 |
type VI secretion system Vgr family protein |
-2.03 |
XOO0079 |
hpaA;HpaA |
-2.59 |
XOO0844 |
protease |
-2.03 |
XOO1822 |
hypothetical protein |
-2.59 |
XOO1259 |
peptidase |
-2.03 |
XOO4535 |
RTS beta protein |
-2.60 |
XOO0094 |
hrp 1 |
-2.05 |
XOO3277 |
hypothetical protein |
-2.62 |
XOO2522 |
hypothetical protein |
-2.05 |
XOO0094 |
hrcC;HrcC |
-2.62 |
XOO4431 |
cirA;TonB-dependent receptor |
-2.07 |
XOO1157 |
pilM;fimbrial assembly membrane protein |
-2.62 |
XOO1943 |
nodQ;ATP sulfurylase/adenylylsulfate kinase |
-2.07 |
XOO2231 |
proP;Prop transport protein |
-2.63 |
XOO3773 |
nrtB;permease |
-2.08 |
XOO4418 |
salR;sal operon transcriptional repressor |
-2.66 |
XOO2294 |
phosphatase |
-2.10 |
XOO3464 |
Type I restriction enzyme StySPI specificity protein |
-2.67 |
XOO3594 |
rplA;50S ribosomal protein L1 |
-2.13 |
XOO1831 |
histidine kinase-response regulator hybrid protein |
-2.68 |
XOO0495 |
radC;DNA repair protein RadC |
-2.15 |
XOO3372 |
thiG;thiazole synthase |
-2.68 |
XOO2348 |
hypothetical protein |
-2.16 |
XOO0019 |
;hypothetical protein |
-2.69 |
XOO3127_ |
hypothetical protein |
-2.17 |
XOO0093 |
hrpB8;HrpB8 |
-2.69 |
XOO3184 |
rplT;50S ribosomal protein L20 |
-2.17 |
XOO0704 |
hypothetical protein |
-2.72 |
XOO0348 |
;pseudouridylate synthase |
-2.18 |
XOO3238 |
tpiA;triosephosphate isomerase |
-2.73 |
XOO0408 |
csd2 family |
-2.18 |
XOO2744 |
xrvA;virulence regulator |
-2.75 |
XOO4263 |
icfG;IcfG protein |
-2.19 |
XOO1077 |
Cellulase S |
-2.75 |
XOO2561 |
;hypothetical protein |
-2.20 |
XOO3557 |
rplQ;50S ribosomal protein L17 |
-2.76 |
XOO3449 |
uidR;transcriptional regulator uid family |
-2.20 |
XOO3269 |
integral membrane protein |
-2.78 |
XOO0869 |
CRISPR-associated proteins |
-2.21 |
XOO2084 |
tRNA/rRNA methyltransferase |
-2.78 |
XOO4265 |
SpolI AA |
-2.22 |
XOO3000 |
cytochrome C |
-2.83 |
XOO1497 |
cysteine protease |
-2.23 |
XOO3255 |
truA;tRNA pseudouridine synthase A |
-2.85 |
XOO0705 |
oxidoreductase |
-2.23 |
XOO1456 |
hypothetical protein |
-2.85 |
XOO2530 |
Dehydrogenases |
-2.26 |
XOO1590 |
fic;Cell filamentation protein Fic |
-2.85 |
XOO0409 |
TolB protein |
-2.26 |
XOO4465 |
hypothetical protein |
-2.87 |
XOO1612 |
hypothetical protein |
-2.27 |
XOO4426 |
hypothetical protein |
-2.87 |
XOO1908 |
hypothetical protein |
-2.29 |
XOO0174 |
hypothetical protein |
-2.89 |
XOO1262 |
;hypothetical protein |
-2.30 |
XOO0411 |
hypothetical protein |
-2.90 |
XOO0407 |
piuB;iron-uptake factor |
-2.31 |
XOO4490 |
hypothetical protein |
-2.91 |
XOO1201 |
omp21;outer membrane protein |
-2.31 |
XOO2127 |
avirulence protein, Avr/PthA |
-2.92 |
XOO0028 |
kdgK;2-keto-3-deoxygluconate kinase |
-2.31 |
XOO0703 |
rsuA;ribosomal small subunit pseudouridylate synthase |
-2.92 |
XOO0747 |
hypothetical protein |
-2.33 |
XOO3561 |
rpsM;30S ribosomal protein S13 |
-2.96 |
XOO1606 |
hypothetical protein |
-2.34 |
XOO2746 |
hypothetical protein |
-2.96 |
XOO2499 |
phy;putative phytase precursor |
-2.35 |
XOO0288 |
transglycolase; epimerase |
-2.99 |
XOO2989 |
methyltransferase |
-2.35 |
XOO1531 |
phuR;outer membrane hemin receptor |
-3.00 |
XOO2346 |
hypothetical protein |
-2.35 |
XOO1160 |
pilP;fimbrial assembly protein |
-3.05 |
XOO2147 |
cycH;C-type cytochrome biogenesis protein |
-2.38 |
XOO2986 |
glycosyltransferase |
-3.08 |
XOO2047 |
hypothetical protein |
-2.38 |
XOO1401 |
acetyltransferase |
-3.09 |
XOO3205 |
hypothetical protein |
-2.38 |
XOO1055 |
hypothetical protein |
-3.10 |
XOO1528 |
short chain dehydrogenase |
-2.38 |
XOO2845 |
tsr;chemotaxis protein |
-3.10 |
XOO4482 |
yojM;superoxide dismutase like protein |
-2.39 |
XOO3559 |
rpsD;30S ribosomal protein S4 |
-3.13 |
XOO2263 |
hypothetical protein |
-3.01 |
XOO4527 |
oxidoreductase |
-3.16 |
XOO0947 |
thiC;thiamine biosynthesis protein ThiC |
-3.01 |
XOO0420 |
hypothetical protein |
-3.16 |
XOO3584 |
rplC;50S ribosomal protein L3 |
-3.02 |
XOO4466 |
XopP, Nucleoside hydrolases |
-3.20 |
XOO1994 |
brf;bacterioferritin |
-2.40 |
XOO2022 |
serine peptidase |
-3.21 |
XOO03911 |
prnA;tryptophan halogenase |
-2.42 |
XOO2667 |
hypothetical protein |
-3.22 |
XOO1069 |
Transglutaminase |
-2.44 |
XOO2246 |
;hypothetical protein |
-3.25 |
XOO3281 |
metallopeptidase |
-2.44 |
XOO0077 |
hrpD6;HrpD6 |
-3.31 |
XOO0084 |
hrcV;HrcV |
-2.44 |
XOO3735 |
vanR;transcriptional regulator gntR family |
-3.33 |
XOO3570 |
rplE;50S ribosomal protein L5 |
-2.46 |
XOO4355 |
Dipeptidyl aminopeptidases |
-3.36 |
XOO2688 |
hypothetical protein |
-2.52 |
XOO1541 |
hypothetical protein |
-3.43 |
XOO2042 |
ldp;dihydrolipoamide dehydrogenase |
-2.53 |
XOO3479 |
hcp;hypothetical protein |
-3.47 |
XOO2705 |
efP;elongation factor P |
-2.54 |
XOO4532 |
hypothetical protein |
-3.59 |
XOO3566 |
rpsE;30S ribosomal protein S5 |
-2.54 |
XOO0008 |
tonB;TonB protein |
-3.63 |
XOO0699 |
;hypothetical protein |
-2.55 |
XOO3410 |
methyltransferase |
-3.64 |
Type II secretory system related genes
We found that hrpXo regulates T2SS effector genes, including 4 cellulase genes (XOO4036, XOO1077, XOO2699, and XOO4019), 6 protease genes (XOO0007, XOO1497, XOO0387, XOO1480, XOO1487, and XOO0844), a polygalacturonase gene (XOO2699), and a lipase gene (XOO0526). Cell-wall degrading enzymes, such as cellulases, pectinases, xylanases, and proteases, are secreted by plant pathogen cells to break down the components of host cell walls and may play a crucial role in virulence and bacterial nutrition (Barras et al. 1994; Shen and Ronald 2002). Currently, genes encoding celldegrading enzymes (CDEs) such as cellulase and xylanase are considered to play a role in the virulence of Xoo (Hu et al. 2007; Rajeshwari et al. 2005; Ray et al. 2000; Sun et al. 2005; Wang et al. 2008). Moreover, this study showed that hrpXo regulates the transcriptional expression of cellulase genes in Xoo. It has been reported that purified cellulase and lipase proteins induce defense responses in rice that are suppressible by Xoo in a T3S-dependent manner (Jha et al. 2007). Therefore, cellulase genes are considered to participate in diverse virulence functions associated directly or indirectly with the expression of the main pathogenicity-related genes, such as hrp genes. In the case of other Xanthomonas species, 2 pathogenicity-related genes (pghAxc and pghBxc) encoding functional polygalacturonase (PG) from X. campestris pv.
campestris 8004 are regulated by HrpX (Wanga), et al. 2008). It was also reported that hrpX negatively regulates the α-amylase isozymes in X. axonopodis pv. citri (Yamazaki et al. 2008) and extracellular proteases in X. campestris pv. campestris (Wei et al. 2007). In a previous study conducted using SDS-PAGE and RT-PCR analysis, hrpXo was shown to regulate the expression of genes associated with T2S proteins, i.e., 2 cysteine proteases (Cysp1 and Cysp2) (Furutani et al. 2004), which shows good agreement with our results. In other phytopathogenic bacteria, it is known that the TTSS effectors encoding cysteine proteases, such as XopD, AvrXv4, AvrPphB, and AvrRpt2, proteolyze specific host targets and are indirectly recognized by R proteins as a consequence of their action in plant cells (Andrew and Mary 2004). Thus, it can be reasonably assumed that hrpXo-dependent cysteine proteases may function as T3SS effectors or indirectly control the recognition of the host plant.
Bacterial mobility
On the basis of the microarray data obtained in this study, it was revealed that hrpXo regulates pilMNEGIP, which encodes fimbrial as asembly proteins that are known as type IV pili. Type IV pili are flexible, filamentous structures protruding from the cell surface of gram-negative bacteria (Shi and Sun 2002; Strom andLory 1993). It has been revealed that type IV pili promote the attachment of bacterial pathogens
to the specific receptors of host cells during colonization (Bieber et al. 1998). Such attachment is an essential event for the initiation of infection. Limited to colonizing the water-conducting xylem vessels of plants, phytopathogenic bacteria develop biofilms that contribute to the blockage of sap flow and thereby cause plant stress and disease (Maxwell et al. 2003; Meng et al. 2005). How bacteria are disseminated in the xylem vessels from feeding sites is an important question. Generally, it is known that type IV pili in bacteria play an important role in twitching and social gliding motility for cell movement (Mattick 2002). On the basis of colony morphologies, there is also strong evidence for a functional role of pil genes in twitching motility in the phytopathogenic bacteria Ralstonia solanacearum and X. fastidiosa (Liu et al.2002; Meng et al.2005). The distribution of pili genes in the Xoo genome was analyzed according to the Xoo sequence information released on the NCBI website. Interestingly, 26 pili-related genes were present in different regions of the Xoo genome, including pilA, pilZ, pilH, pilMNOPQ, pilTU, pilDCE, pilBRS, pilGHIJL, pilF, pilE1Y1, pilX, and pilV (Lee et al. 2005). Xoo genome analysis suggests that a number of pili gene orthologs may encode proteins involved in the biogenesis and function of type IV pili. However, the functional roles of other pili genes have not been proved in the Xoo genome. It was recently reported that Xoo pilQ plays a critical role in pathogenicity, twitching motility, and biofilm production (Lim, et al. 2008). It was previously reported that 14 pili genes (pilIJKLMNOPQRSTUV) are involved in type IV pilus biogenesis in E. coli (Sakai andKomano 2002). The genes pilS, pilV, and pilU encode the major pilin, minor pilin, and prepilin peptidase, respectively. The C-terminal segments of pilV genes are under the control of multiple DNA inversions of shufflon and determine recipient specificity in liquid mating. The pilL and pilN gene products are outer-membrane lipoproteins, and the pilQ gene product is a cytoplasmic ATPase and is essential for thin pilus formation (Saiki et al. 2001). The remaining pil genes are likely to
encode structural proteins that function in the establishment of the pilin transport apparatus and the basal body of the thin pilus. Most of these pil gene products contain signal sequences or trans-membrane domains, suggesting that they are transported to the peri-plasmic space, inner membrane, and outer membrane.
Verification of microarray results
The effect of the hrpXo mutation on the expression of different genes was validated by using RT-PCR. The RT-PCR 20-mer primers were generated from 12 T3SS-related hrp genes, 4 T2SS-related cysteine protease-encoding genes, 3 cellulase genes, and 6 type-IV pili genes. As shown in Fig. 2, no, or very weak, amplification was obtained from the genes in the hrpXo::Tn5 mutant, although the magnitude of expression was somewhat different to those observed in the microarray analysis. As expected, all hrp gene primers tested amplified RT-PCR bands with reduced intensity in the hrpXo::Tn5 mutant, compared with the intensities for the wild-type strain. In addition, 4 genes encoding cysteine proteases and cellulases (XOO4036) did not amplify an RT-PCR band in the hrpXo::Tn5 mutant, indicating that their transcriptional expression is absolutely dependent on hrpXo. Furthermore, RT-PCR analysis also verified that when compared with the wild-type strain, the hrpXo mutant visually represents low transcriptional expression of pilE, pilG, pilI, and pilP, but not pilM and pilN. The result confirmed that the microarray result is largely available.
In conclusion, DNA microarray analysis of X. oryzae pv. oryzae revealed that in addition to regulating the hrp genes, the hrpX regulon regulates numerous new genes possessing different functions. Furthermore, the potential interactive roles between the hrpX regulons and the host plant should be elucidated for pathogenesis mechanisms.
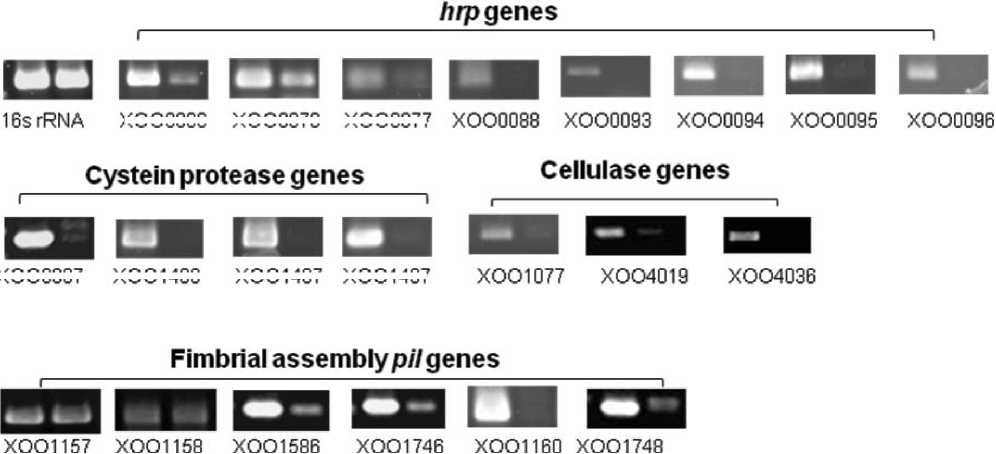
XOO0387
XOO0066 XOO0076 XOO0077
XOO1480 XOO1487 XOO1497
Fig.3. Verification of microarray data by RT-PCR. Total RNAs from wild-type strain KACC10859 and the hrpX:: TN mutant were used as PCR templates with primer pairs in Table 2. Amplicons were visualized after agarose gel electrophoresis and ethidium bromide staining.
REFERENCES
Andrew H, Mary BM. 2004. Cysteine proteases in phytopathogenic bacteria: identification of plant targets and activation of innate immunity. Current Opinion in Plant Biology 7: 1-7
Astua-Monge G, Freitas-Astua J, Bacocina G , Roncoletta J, Carvalho SA, Machado MA. 2005.Expression profiling of virulence and pathogenicity genes of Xanthomonas axonopodis pv. citri. J. Bacteriol. 187: 12011205.
Barras F. 1994. Exreacellular enzymes and pathogenesis of soft-rot Erwinia. Ann Rev Phytopathol. 32: 201-234
Bieber D, Ramer SW, Wu CY, Murray WJ, Tobe T, Fernandez R, Schoolnik GK. 1998. Type IV pili, transient bacterial aggregates, and virulence of enteropathogenic Escherichia coli. Science 280: 2114-2118.
Bu¨ttner D, Bonas U. 2002. Getting across— bacterial type III effector proteins on their way to the plant cell. EMBO J. 21: 5313– 5322.
Chan JW, Goodwin PH. 1999. The molecular genetics of virulence of Xanthomonas campestris. Biotechnol. advance 17: 489-580
da Silva ACR and Ferro JA. 2002. Comparison of genomes of two Xanthomonas pathogens with differing host specificities. Nature 417: 459-463.
de Morgan A, Brodsky LY, Ronin Y, Nevo E, Korol A, Kashi, Y. 2010. Genome-wide analysis of DNA turnover and gene expression in stationary-phase Saccharomyces cerevisiae. Microbiol 156: 1758–1771
Feng JX, Song ZZ, Duan CJ, Zhao S, Wu YQ, Wang C, J. Dow M, Tang JL. 2009. The xrvA gene of Xanthomonas oryzae pv. oryzae, encoding an H-NS-like protein, regulates virulence in rice. Microbiol. 155: 3033-3044
Furutani A, Nakayama, T, Ochiai, H, Kaku H, Kubo Y, Tsuge S. 2006. Identification of novel HrpXo regulalons preceded by two cis-acting elements, a plant-inducible promoter box and a -10 box-like sequence, from the genome database of Xanthomonas oryzae pv. oryzae. FEMS Microbiol Letter 259: 133-141
Furutani A, Takaoka M, Sanada H, Noguchi Y, Oku T, Tsuno K, Ochiai H, Tsuge S. 2009. Identification of Novel Type III Secretion Effectors in Xanthomonas oryzae pv. oryzae. Mol. Plant- Microbe Interact. 22: 96–106
Furutani A, Tsuge S, Ohnishi K, Hikichi Y, Oku T, Tsuno, K, Inoue Y, Ochiai H, Kaku H, Kubo Y. 2004. Evidence for HrpXo-dependent expression of type II secretory proteins in Xanthomonas oryzae pv. oryzae. J. Bacteriol. 186: 1374–1380.
Ghindilis AL, Smith MW, Schwarzkopf KR, Roth KM, Peyvan,K, Munro SB, Lodes MJ, St¨over AG, Bernards K, Dill K, McShea A. 2007. CombiMatrix oligonucleotide arrays: Genotyping and gene expression assays employing electrochemical detection. Biosens. Bioelectro. 22: 1853–1860
Grulebeck D, Thieme F, Bonas U. 2005. Type III effector proteins from the plant pathogen Xanthomonas and their role in the interaction with the host plant. J. Plant Physiol.163: 233-255.
Gu K, Yang B, Tian D, Wu L, Wang D, Sreekala C, Yang F, Chu Z, Wang GL, White FF, Yin Z. 2005. R gene expression induced by a type-III effector triggers disease resistance in rice. Nature 435: 1122-1125.
Gustavo AM, Juliana FA, Gisele B, Juliana R, Se´rgio AC, Marcos AM. 2005. Expression Profiling of Virulence and Pathogenicity Genes of Xanthomonas axonopodis pv. citri. J. Bacteriol. 187: 1201-1205
Guidot A, Coupat B, Fall S, Prior P, Bertolla F. 2009. Horizontal gene transfer between Ralstonia solanacearum strains detected by comparative genomic hybridization on microarrays. The ISME Journal. 3: 549-562 He YQ, Zhang L, Jiang BL, Zhang ZC, Xu RQ, Tang DJ, Qin J, Jiang W, Zhang X, Liao J, Cao JR, Zhang SS, Liang XX, Wei ML, Lu GT, Feng JX, Chen B, Cheng J, Tang JL. 2007. Comparative and functional genomics reveals genetic diversity and determinants of host specificity among reference strains and a large collection of Chinese isolates of
the phytopathogen Xanthomonas campestris pv. campestris. Genome Biol. 8: R218.
He YW, Xu M, Lin K, Ng YJ, Wen CM, Wang LH, Liu ZD, Zhang HB, Dong YH, Dow JM, Zhang LH. 2006. Genome scale analysis of diffusible signal factor regulon in Xanthomonas campestris pv. campestris: identification of novel cellcell communicationdependent genes and functions. Mol. microbial. 59: 610-622.
Hu J, Qian W, He CZ. 2007. The Xanthomonas oryzae pv. oryzae eglXoB endoglucanase gene is required for virulence to rice. FEMS Microbiol letter 269: 273-279.
Jha G, Rajeshwari R, Sonti RV. 2007. Functional interplay between two Xanthomonas oryzae pv. oryazae secretion systems in modulating virulence on rice. Mol. Plant- Microbe Interact. 20: 31-40.
Koebnik R, Kruger A, Thieme F, Urban A, Bonas U. 2006. Specific binding of the Xanthomonas campestris pv. vesicatoria AraC-type transcriptional activator HrpX to plantinducible promoter boxes. J. Bacteriol. 188: 7652-7660.
Koide T, Vencio RZ, Gomes SL. 2006. Global gene expression analysis of the heat shock response in the phytopathogen Xylella fastidiosa. J. Bacteriol. 188: 5821-5830.
Kuhnert P, Heyberger-Meyer B, Burnens AP, Nicolet J, Frey J. 1997. Detection ofRTXtoxin genes in gram-negative bacteria with a set of specific probes. Appl. Environ. Microbiol. 63: 2258–2265.
Lee BM, Park YJ, Park DS, Kang HW et al. 2005. The genome sequence of Xanthomonas oryzae pathovar oryzae KACC10331, the bacterial blight pathogen of rice. Nuc. Acids Res. 33: 577-586.
Lim SH, So BH, Park YJ, Lee BM, Kang HW. 2008. Functional analysis of pilQ gene in Xanthomanas oryzae pv.oryzae. J. Microbiol. 46: 214-220.
Liu HL, Kang YW, Genin S, Schell MA, Denny TP. 2001. Twitching motility of Ralstonia solanacearum requires a type IV pilus
system. Microbiol. 147: 3215-3229.
Lu SE, Wang N, Wang J, Chen ZJ, Gross DC. 2005. Oligonucleotide microarray analysis of the salA regulon controlling phytotoxin production by Pseudomonas syringae pv. syringae. Mol. Plant Microbe Interact. 18: 324-333.
Mattick, JS. 2002. Type pili and twitching motility. Annu. Rev. Microbiol. 56: 289-314.
Maxwell JL, Crossman K, Findlay YQ, He J, Feng X, Tang JL. 2003. Biofilm dispersal in Xanthomonas campestris is controlled by cell-cell signaling and is required for full virulece to plants. Proc. Natl. Acad. Sci. 100: 10995-11000.
Meng YY, Li Y, Galvani CD, Hao G, Turner JN, Burr TJ, Hoch HC. 2005. Upstream migration of Xylella fastidiosa via pilus-driven twitching motility. J Bacteriol. 187: 5560-5567.
Noe¨l L, Thieme F, Ninnstiel D and Bonas U. 2001. cDNA-AFLP analysis unravels a genome-wide hrpG-regulon in the plant pathogen Xanthomonas. Mol Microbiol 41: 1271–1281
Ochiai H, Inous Y, Takeya M, Sasaki A and Kaku H. 2005. Genome sequence of Xanthomonas oryzae pv. oryzae suggests contribution of large numbers of effecter genes and insertion sequences to its race diversity. Jap.Agri. Res. 39: 275-287.
Okazaki S, Sugawara M, Minamisawa K. 2004. Bradyrhizobium elkanii rtxC Gene Is Required for Expression of Symbiotic Phenotypes in the FinalStepofRhizobitoxineBiosynthesis. Appl Environ. Microbiol. 70: 535-541
Oku T, Alvarez AM, Kado CI. 1995. Conservation of the hypersensitivity-pathogenicity regulatory gene hrpX of Xanthomonas campestris and X. oryzae. DNA Seq. 5: 245– 249.
Oresnik IJ, Twelker S, Hynes MF. 1999. Cloning and characterization of a Rhizobium leguminosarum gene encoding a bacteriocin with similarities to RTX toxins. Appl. Environ. Microbiol. 65: 2833–2840.
Park YJ, Song ES, Kim YT, Noh TH, Kang HW, Lee BM. 2007. Analysis of virulence and growth ofa purine auxotrophic mutant of Xanthomonas oryzae pathovar oryzae. FEMS Microbiol. Letter 276: 55-59
Rajeshwari R, Jha G, Sonti V. 2005. Role of an In Planta-expressed xylanase of Xanthomonas oryzae pv.oryzae in promoting virulence on rice. Mol Plant Microbe Interact. 18: 830837
Ray S, Rajeshwari R, Sonti RV. 2000. Mutants of Xanthomonas oryzae pv.oryzae deficient in general secretory pathway are virulence deficient and unable to secret xylanase. Mol Plant Microbe Interact. 13: 394-401
Sakai D, Komano T. 2002. Genes required for plasmid R64 thin-pilus biogenesis: identification and localization of products of the pilK, pilM, pilO, pilP, pilR, and pilT genes. J. Bacteriol. 184: 444-452
Sakai D, Horiuchi T, Komano T. 2001. ATPase activity and multimer formation of PilQ protein are thin pilus biogenesis in plasmid R64. J. Bio.Chem. 276: 17968-17975
Seo YS, Sriariyanun M, Wang L, Pfeiff J, Phetsom J, Lin Y, Jung KH, Chou HH, Bogdanove A, Ronald P. 2008. A two-genome microarray for the rice pathogens Xanthomonas oryzae pv. oryzae and X. oryzae pv. oryzicola and its use in the discovery of a difference in their regulation of hrp genes. BMC microbial. 8: 86-99
Simpson AJ, Reinach FC, Arruda P, Abreu FA, Acencio M, Alvarenga R, Alves LM, Araya JE, Baia GS, Baptista CS, Barros MH. 2000. The genome sequence of the plant pathogen Xylella fastidiosa. Nature 406: 151–157.
Shen Y, Ronald P. 2002. Molecular determinants of disease and resistance in interactions of Xanthomonas oryzae pv. oryzae and rice. Microbes Infect. 4: 1361-1367
Shi W, Sun H. 2002. Type IV Pilus-Dependent Motility and Its Possible Role in Bacterial Pathogenesis. Infection immunol 70: 1–4.
Strom MS, Lory S. 1993. Structure function and biogenesis of the type IV pili. Annu. rev.
Microbiol 47: 565-597.
Sun QH, Hu J, Huang GX, Ge C, Fang RX, He CZ. 2005. Type-II secretion pathway structural gene xpsE, xylanase- and celllulase secretion and virulence in Xanthomonas oryzae pv. oryzae. Plant Pathol. 54: 15-21
Tsuge S, Furutani A, Fukunaka R, Oku T, Tsuno K, Ochiai H, Inoue Y, Kaku H, Kubo Y. 2002. Expression of Xanthomonas oryzae pv. oryzae hrp genes in a novel synthetic medium, XOM2. J. Gen. Plant Pathol. 68: 363–371.
Tsuge S, Nakayama T, Terashima S, Ochiai H, Furutani A, Oku T, Tsuno K, Kubo Y, Kaku H. 2006. Gene Involved in Transcriptional Activation of the hrp Regulatory Gene hrpG in Xanthomonas oryzae pv. oryzae. J. Bacteriol. 188: 4158-4162
Tsuge S, Terashima S, Furutani A, Ochiai H, Oku T, Tsuno K, Kaku H, Kubo Y. 2005. Effects on Promoter Activity of Base Substitutions in the cis-Acting Regulatory Element of HrpXo Regulons in Xanthomonas oryzae pv. oryzae. J. Bacteriol. 187: 2308-2314
Wang L, Wei R, He C. 2008. Two Xanthomonas extracellular polygalacturonases, PghAxc and PghBxc, are regulated by type III secretion regulators HrpX and HrpG and are required for virulence. Mol Plant Microbe Interact. 21: 555–563.
Wang JC, So BH, Lee DS, Park YJ, Lee BM, Kang HW. 2008. Genome Wide- Identification of virulence Genes in Xanthomonas oryzae pv. oryzae by Transposon Mutagenesis. Plant Pathol. 57: 1136-1145
Wang N, Lu SE, Wang J, Chen ZJ, Gross DC. 2006. The expression of genes encoding lipodepsipeptide phytotoxins by Pseudomonas syringae pv. syringae is coordinated in response to plant signal molecules. Mol Plant Microbe Interact. 19: 257-269.
Welch R, Forestier A, Lobo C, Pellett A, Thomas SW, Rowe G. 1992. The synthesis and function of the Escherichia coli hemolysins and related RTX exotoxins. FEMS Microbiol
Immunol. 105: 29–36.
Wei K, Tang DJ, He YQ, Feng JX, Jiang BL, Lu GT, Chen B, Tang JL. 2007. hpaR, a Putative marR Family Transcriptional Regulator, Is Positively Controlled by HrpG and HrpX and Involved in the Pathogenesis, Hypersensitive Response,andExtracellular Protease Production of Xanthomonas campestris pathovar campestris. J. Bacteriol. 189: 2055-2062
Wengelnik K, Bonas U. 1996. HrpXv, an AraC-type regulator, activates expression of five of six loci in the hrp cluster of Xanthomonas campestris pv. vesicatoria essential for pathogenicity and induction of the hypersensitive reaction. J. Bacteriol. 178: 3462–3469.
Wengelnik K, Marie C, Russel M, Bonas U. 1996. Expression and localization of HrpA1, a protein of Xanthomonas campestris pv. vesicatoria essential for pathogenicity and induction of the hypersensitive reaction. J. Bacteriol. 178: 1061–1069.
Wengelnik K, Rossier O, Bonas U. 1999. Mutations in the regulatory gene hrpG of Xanthomonas campestris pv. vesicatoria result in constitutive expression of all hrp genes. J. Bacteriol. 181: 6828–6831.
Wiggerich HG, Klauke B, Koplin R, Priefer UB, Puhler A. 1997. Unusual structure of the tonB-exb DNA region of Xanthomonas campestris pv. campestris: tonB, exbB, and exbD1 are essential for ferric iron uptake, but exbD2 is not. J. Bacteriol. 179: 71037110
Wiggerich HG, Puhler A. 2000. The exbD2 gene as well as the iron-uptake genes tonB, exbB and exbD1 of Xanthomonas campestris pv. campestris are essential for the induction of a hypersensitive response on pepper (Capsicum annuum). Microbiol. 146: 1053– 1060
Wu J, Wu M, He C. 2010. Identification and functional analysis of Tdrxoo, the member of TonB-dependent-receptor family proteins in Xanthomonas oryzae pv. oryzae.
Wei Sheng Wue Bao 50: 155-161
Yamazaki A, Hirata H, Tsuyumu S. 2008. Type III regulators HrpG and HrpXct control synthesis of α-amylase, which is involved in planta multiplication of Xanthomonas axonopodis pv.citri. J. Gen. Plant Pathol. 74: 254–257.
Yang B, Zhu W, Johnson LB, White FF. 2000. The virulence factor AvrXa7 of Xanthomonas oryzae pv. oryzae is a type III secretion pathway-dependent nuclear-localized double-stranded DNA-binding protein. Proc Natl Acad Sci. U.S.A. 97: 9807-9812.
20 • udayana university biosciences and biotechnology forum
Discussion and feedback